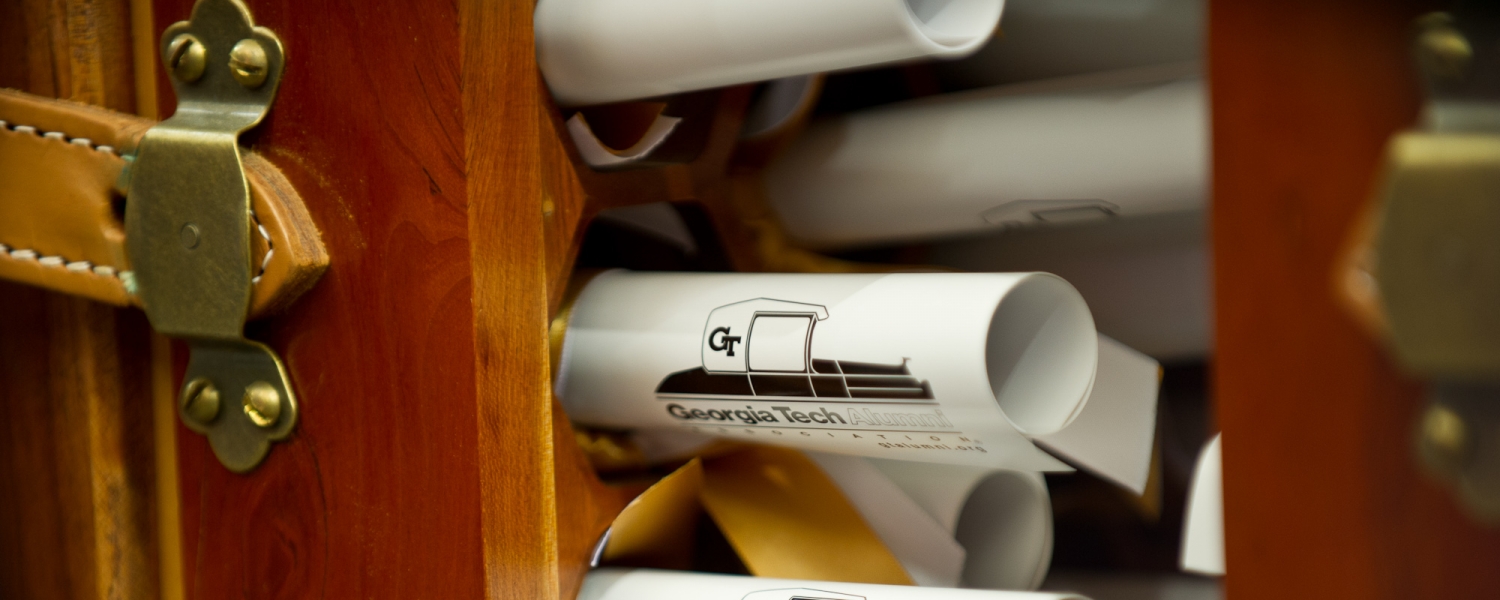
Abstract
Scalable quantum computing fundamentally relies on quantum error correction and fault-tolerance. Sustained efforts in the engineering of controllable quantum systems and high-fidelity quantum measurement have driven these theoretical concepts into the domain of practical experimental science. In this talk, I will present evidence that the physics of quantum error correction is also a generic, emergent property of quantum many-body systems subject to continuous monitoring of their environment. Using toy models based on random unitary dynamics interspersed with projective measurements, I will describe precise connections that arise between fundamental notions of quantum error correction, such as the channel capacity, and the existence of a phase transition in these models as one tunes the measurement rate. I will then show how to define a local order parameter for this transition that is defined by the ability of the system to protect initially local quantum information for exponentially long times. This construction points to scalable probes of the transition that are immediately applicable to quantum computing platforms such as trapped ions or superconducting qubits. Studying this class of measurement-driven many-body dynamics may ultimately lead to more efficient realizations of scalable, fault-tolerant quantum computing, as well as deepen our understanding of the transition from quantum to classical physics in many-body systems.
Event Details
Date/Time:
With energies greater than a PeV, cosmic rays from galactic (and extra-galactic) sources are too rare to detect directly. Instead, large ground-based detector arrays catch the secondary particles from the extensive air showers that cosmic rays produce in the atmosphere, and attempt to determine indirectly the underlying spectrum and mass composition. The IceCube Observatory has a surface detector called IceTop, which can help to answer these questions... questions which are linked to the mystery of these particles' origin.
Event Details
Date/Time:
Abstact
The concept of trophic level comes from ecosystems where the idea was to quantify how many steps in a food chain a species is from the energy source. The catch is that given a preliminary classification into integer levels, one quickly finds that some species eat things from more than one level. A real-valued notion of trophic level was proposed by Levine to take this into account and has become the accepted standard. The notion was rediscovered by economists in supply networks under the name “upstreamness”. It has been applied to many other classes of directed network.
Johnson et al introduced the notion of trophic coherence, measuring the standard deviation of the trophic height differences along the edges in a network, and showed it to be a good predictor of many other network properties, such as stability of contagion processes, numbers of cycles, and non-normality.
Traditional trophic analysis suffers from three defects, however. Firstly, it is over-sensitive to what is included as basal species and cannot be implemented at all if there are none. Secondly, the results are not equivariant with respect to reversing all the edges. Thirdly, it does not allow a natural way to do local trophic analysis.
Our trophic analysis remedies all three defects. The improved trophic levels give useful information and the improved notion of trophic coherence has similar relations to network properties. A special mention will be made about zeta functions for networks.
Event Details
Date/Time:
Abstract
Collective behavior of organisms creates environmental micro-niches that buffer them from environmental fluctuations e.g., temperature, humidity, mechanical perturbations, etc., thus coupling organismal physiology, environmental physics, and population ecology. This talk will focus on a combination of biological experiments, theory, and computation to understand how a collective of bees can integrate physical and behavioral cues to attain a non-equilibrium steady state that allows them to resist and respond to environmental fluctuations of forces and flows.
We analyze how bee clusters change their shape and connectivity and gain stability by spread-eagling themselves in response to mechanical perturbations. Similarly, we study how bees in a colony respond to environmental thermal perturbations by deploying a fanning strategy at the entrance that they use to create a forced ventilation stream that allows the bees to collectively maintain a constant hive temperature. When combined with quantitative analysis and computations in both systems, we integrate the sensing of the environmental cues (acceleration, temperature, flow) and convert them to behavioral outputs that allow the swarms to achieve a dynamic homeostasis.
Event Details
Date/Time:
Abstract
Artificial control of animal locomotion has the potential to address previously inaccessible questions about the biology of swimming organisms and animal-fluid interactions, where we are otherwise limited to observations of natural behavior. This work presents a biohybrid robot that uses a self-contained microelectronic system to induce swimming in live jellyfish. By driving body contractions at an optimal frequency range faster than observed in natural behavior, swimming speed can increase nearly threefold, with only a twofold increase in cost of transport to the animal. Furthermore, this robotic system uses 10 to 1000 times less external power per mass than existing swimming robots in literature. This capability can potentially be leveraged in applications such as ocean monitoring, and to enable further studies of swimming organisms in more user-controlled, systematic experiments.
Event Details
Date/Time:
Abstract
I will discuss how control over light-matter interactions can be used for simulating fundamental physics in optical table-top experiments and developing novel theoretical and experimental tools for photonic large-scale entanglement generation. Specifically, I will first describe the experimental simulation of the Newton-Schrödinger system, a nonlinear gravity model, in a system of long-range-interacting optical wavepackets by thermal nonlinearity. I will next describe the novel concept of atomic quantum metasurfaces, a new interface for quantum light-matter interaction. Specifically, I will show how these quantum metasurfaces can be realized in realistic systems and how they can be used for generation of large-scale atom-photon entanglement. I will discuss recent experimental developments and possible new applications. Finally, I will discuss our ongoing efforts to realize large-scale entanglement with atom-like defects in nanophotonic cavities.
Event Details
Date/Time:
Superconducting circuits have emerged as one of the most promising platforms for quantum computation as a result of rapid advances in coherence and control over the past few decades. Most modern superconducting processors are based on the transmon circuit, and rely on nearest-neighbor interactions for gate operations and entanglement. In this talk, I will present an alternative architecture for superconducting quantum information and simulation, involving many harmonic modes of a multimode cavity coupled and controlled by a single transmon circuit. This multimode circuit-QED system leverages the long coherence times and restricted decoherence channels of superconducting microwave cavities. Additionally, the architecture has a high degree of connectivity while being hardware efficient, with gate operations performed between arbitrary pairs of cavity modes using only a few control lines that drive the transmon. Our implementation of such a processor uses - the quantum flute, a novel rectangular 3D multimode cavity with a tailored mode dispersion, that is protected from seam loss and possesses O(10) distinct modes with photon lifetimes approaching a millisecond. I will present various schemes for universal control of the multimode Hilbert space using the dispersively coupled transmon, and discuss schemes for engineering designer photon-photon interactions.
Event Details
Date/Time:
We are in the midst of a second quantum revolution in which the laws of quantum mechanics are being applied to gain information processing and computational advantage. However, in order to accomplish tasks that are well beyond the reach of CMOS-based, von Neumann computers operating today, quantum-logic operations must be performed with ultrahigh degree of accuracy. Therefore eventual useful application of quantum technology will absolutely require efficient means of correcting hardware and software errors. The key idea behind quantum error correction (QEC) is that physical noise is local and hence errors are diagnosed by periodically measuring non-local correlations in an extended system. Remarkably, with QEC it becomes possible to carry out arbitrarily accurate quantum computation even with faulty hardware provided the error rate is below some constant threshold. Unfortunately, this tolerance to faults comes at the cost of dauntingly large hardware overhead. Recently, an alternate hardware efficient approach for QEC, based on storing quantum information in oscillators or bosonic modes, is gaining momentum. In this talk, I will present an elementary introduction to QEC as well as introduce some of the recent work in the direction of bosonic QEC.
Event Details
Date/Time:
Abstract
Animal behavior is often quantified through subjective, incomplete variables that may mask essential dynamics. Here, we develop a behavioral state space in which the full instantaneous state is smoothly unfolded as a combination of short-time posture dynamics. Our technique is tailored to multivariate observations and extends previous reconstructions through the use of maximal prediction. Applied to high-resolution video recordings of the roundworm C. elegans, we discover a low-dimensional state space dominated by three sets of cyclic trajectories corresponding to the worm’s basic stereotyped motifs: forward, backward, and turning locomotion. In contrast to this broad stereotypy, we find variability in the presence of locally-unstable dynamics, and this unpredictability shows signatures of deterministic chaos: a collection of unstable periodic orbits together with a positive maximal Lyapunov exponent. The full Lyapunov spectrum is symmetric with positive, chaotic exponents driving variability balanced by negative, dissipative exponents driving stereotypy. The symmetry is indicative of damped, driven Hamiltonian dynamics underlying the worm’s movement control.
Event Details
Date/Time:
Living cells and tissues are highly mechanically sensitive and active. Mechanical forces and stimuli influence the shape, motility, and functions of cells, modulate the behavior of tissues, and play a key role in diseases as different as osteoarthritis and cancer metastasis. In this talk, I will discuss how collective biophysical properties of tissues emerge from the interplay between different mechanical properties and statistical physics of underlying components. I will use examples of two complementary tissue types to illustrate how the emergent mechanobiology of tissues is facilitated by their heterogeneous and composite nature, and proximity to phase transitions. I will start with mechanical structure-function relationships in articular cartilage (AC), a soft tissue that has very few cells, and its mechanical response is primarily due to its network like extra-cellular matrix. AC is a remarkable tissue: it can support loads exceeding ten times our body weight and bear 60+ years of daily mechanical loading and resist fracture, despite having minimal regenerative capacity.
I will discuss the biophysical principles underlying this exceptional mechanical response using the framework of rigidity percolation theory, and compare our predictions with experiments done by our collaborators. Next, I will discuss how differences in cell mechanics, adhesion, and proliferation in a co-culture of breast cancer cells and healthy breast epithelial cells may modulate experimentally observed phase separation and transport properties. Our results may provide insights into the mechanobiology of tissues with cell populations with different physical properties present together, such as during the formation of embryos or the initiation of tumors. By obtaining a mechanistic understanding of the biophysical properties of these two systems, we hope to elucidate principles underlying the robustness and tunability of tissue properties and gain insights into design principles for soft robotics.
Event Details
Date/Time:
Pages
