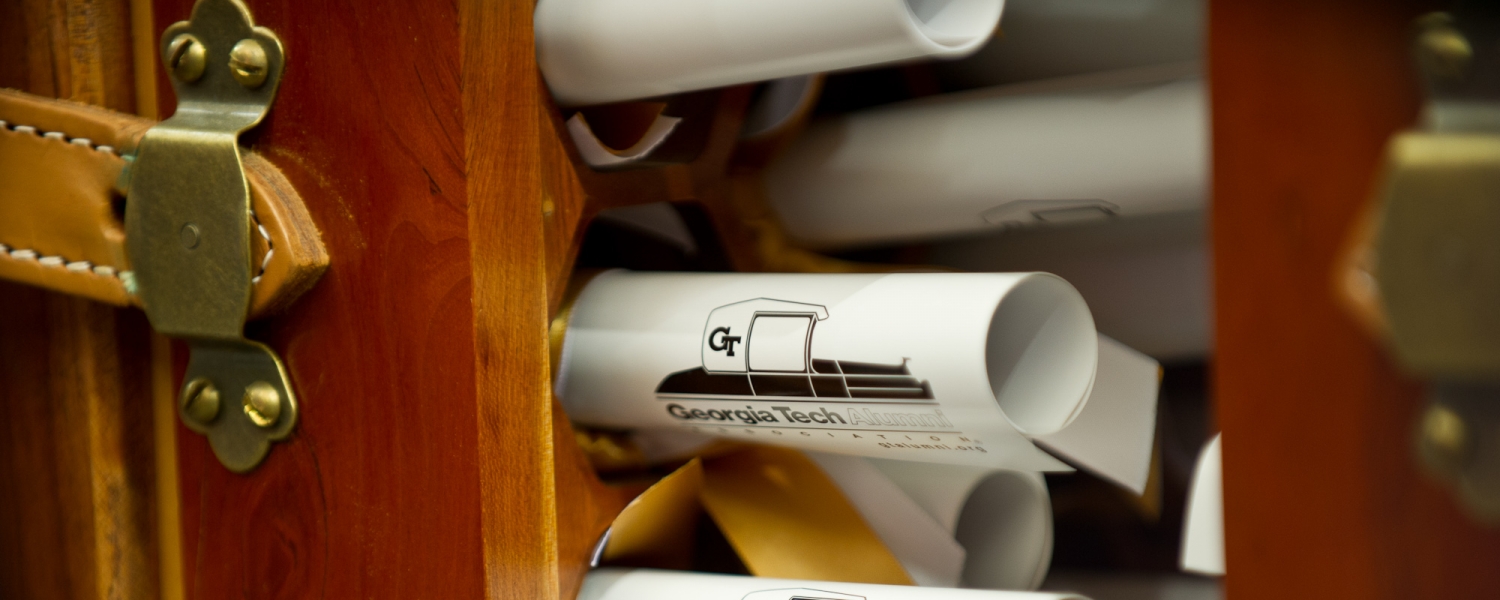
Abstract: Mathematical models have been crucial for understanding biological systems because they help us organize our knowledge about the system and allow us to not only test new ideas without harming or perturbing expensive in vivo, in vitro, or in situ subjects, but to further test new hypothesis. Cardiac electrophysiology is a field that requires a deep understanding of a wide span of physiological scales. From the single-cell ionic membrane exchanges, to the fiber distribution and geometry of the heart. Naturally, this complexity draws several kinds of modelling proposals, many of which describe, with different degrees of complexity, the process of excitation and propagation of an action potential (AP).
In this thesis we will present two model reduction paradigms and the computational tools to use them. First, we introduce a new parsimonious phenomenological model based on the FitzHugh-Nagumo model. We focus on describing its main characteristics and presenting a variety of applications that cover a wide range of subjects. In particular, our model can fit experimental data of several animal species. Moreover, analytical expressions for the restitution and dispersion curves are available. Next, we expand our idea of model reduction by taking advantage of the symmetries of the electrical patterns. We specifically look at translational and rotational invariant solutions. We then present a numerical scheme for symmetry reduction of spiral waves. Afterwards, we tested the method with several models and multiple spiral wave solutions. Finally, we investigated the performance of several parallel programming languages for graphic processing units by comparing the speeds of multiple implementations of a cardiac solver. In this work, we develop the theory and provide the numerical schemes to reproduce our results.
Event Details
Date/Time:
Abstract: Magnetic and nematic phenomena are ubiquitous in nature such as the spontaneous magnetization of magnetite and the formation of liquid crystal. Ultracold atom provides a unique platform for exploring multi-faceted quantum magnetic behavior associated with spin. The interplay between magnetic interaction and the interaction entangling spin and spatial motion (aka spin-orbit coupling) gives rise to rich phase diagrams and phase transitions between different phases, which are ideal tools for understanding universal dynamics in non-equilibrium system. Therefore in my work, we use 23Na spinor Bose-Einstein condensates (BECs) to study two different aspects of nematic and magnetic phenomena, nematic-orbit coupling and magnetic solitons.
On one hand, complex Hamiltonians can be engineered in spinor BEC to simulate condensed matter physics, such as spin-orbit coupling. Spin-orbit coupling mechansim[1], a crucial element in spin-Hall effect, couples the atomic spin to the spatial degree of freedom. As an analogy of spin-orbit coupling, a nematic-orbit coupling[2] mechanism is proposed, which couples the spin-nematic tensor to spatial motion of the atoms. To investigate the nematic-orbit coupling, we come up with an experimental proposal by using coplanar microwave arrays. And the phase diagram is carried out and two new striped phases are found theoretically. This opens a new realm for studying complex spin-nematic objects in spinor BEC.
On the other hand, spinor BEC is a non-linear magnetic system for defects to exist, such as vector solitons. A vector soliton is a type of solitary wave packet occurring in a nonlinear medium comprised of multiple components. In our experiments, a new type of soliton called magnetic solitons[3], is observed in a spinor BEC beyond the usual Manakov limit of the 1-dimensional Gross-Pitaevskii (GP) equations due to the magnetic interactions. By using a phase imprinting technique with magic wavelength, we created a pair of magnetic solitons in an antiferromagnetic spinor BEC. Besides, multiple solitons can be created by spatially modulating the pattern of the phase imprinting beam, which allows the future investigation of the bound state (Flemish strings[4]), which turns out to be a potential candidate accounting for the universal dynamics for the non-equilibrium system generated after a quench from the polar phase to the antiferromagnetic phase of 23Na BEC.
Reference:
[1] Y.-J. Lin, K. Jiménez-García, and I. B. Spielman, Spin–orbit-coupled Bose–Einstein condensates, Nature 471, 83–86 (2011)
[2] D. Lao, C. Raman, and C. A. R. Sa de Melo, Nematic-orbit coupling and Nematic density waves in spin-1 condensates, Phys. Rev. Lett. 124, 173203 (2020)
[3] ] X. Chai, D. Lao, K. Fujimoto, R.Hamazaki, M. Ueda and C. Raman, Magnetic solitons in a spin-1 Bose-Einstein condensate, Phys. Rev. Lett. 125, 030402 (2020)
[4] K. Fujimoto, R. Hamazaki, and M. Ueda, Flemish Strings of Magnetic Solitons and a Nonthermal Fixed Point in a One-Dimensional Antiferromagnetic Spin-1 Bose Gas, Phys. Rev. Lett. 122, 173001 (2019)
Event Details
Date/Time:
Abstract: In movement on solid terrains, animals, vehicles, and robots can make use of well-established contact dynamics for planning movement and locomotion gaits. However, when the terrain can be deformed significantly, effective traversal can be inhibited by terrain heterogeneities created before and/or during locomotion. Understanding the physical behavior of such deformations such complex forcings like locomotion can inform robotic navigation strategies and expand our physical intuition of soft matter substrates. We examine various rate-dependent phenomena for a specific class of flowable substrates abundant in the natural world: granular media, which exhibit multiphase and hysteretic properties as a collective of many small rigid bodies. The physics of granular substrates is dominated by a network of frictional contacts between simple particles, which nevertheless display a wealth of unexpected multiphase phenomena depending on their stresses and packings. We present a series of experimental studies on such media. An anthropogenic mode of terrain traversal, wheeled locomotion, can locomote via the reaction force generated from actively shearing a granular substrate. We experimentally show how such locomotion can induce rate-dependent weakening via the centripetal acceleration of the media under shear and present a fundamental physics-based cause for why vehicular slippage occurs at high wheel rotation rates. In another robophysical study, we demonstrate how a small rover robot can effectively remodel steep granular slopes via strategic open-loop gait selection. By selectively avalanching frictional media towards itself, the robot could traverse loosely consolidated granular hills that otherwise would not be possible for it to climb. Finally, we investigate how directional fluidization of granular media during intrusion could modulate the resistive forces to allow a body to move efficiently within a substrate that constantly attempts frictionally hinder it. This dissertation showcases not only the strength of experimental investigation in physics to discover new phenomena, but also how simple reduced-order models can be adapted to explain them and synthesize our observations for applications in robotics, terradynamics, and more.
Event Details
Date/Time:
Abstract: Like most physical systems, the interaction characteristics among agents play an important role in active matter. For example, the extent of attraction can switch a collective of particles from a homogeneous mixture to phase-separated clusters; particle concavity in shape-changing active systems can change interactions from repulsive to attractive. The way that the force transmits can also be important. While many interactions transmit through direct or short-ranged contact (e.g., collisions or magnetic attraction), there are interactions that require the full description of the force-generating field to describe motion. These interactions can bring interesting features such as time delays, the coexistence of multiple length scales, and non-reciprocity, which are less common in short-ranged interacting systems. In this thesis, I will use several examples from my Ph.D. work to show the rich dynamics of active matter interacting through a field. Examples include active locomotors mimicking motion in curved space-time when driving on an elastic membrane, and resource-consuming agents driven by resource depletion that form different states of matter. Through these studies, I will also show how the connection between field-mediated interactions and classical fields allows us to explain and explore emergent phenomena in active matter using inspiration and tools from field theory. In addition to the study of field-mediated interactions, other studies of active matter with short-ranged interactions are presented in the later chapters. These include shape-changing active matter, the role of substrate curvature in active matter, and the analogy between attractive interaction in active matter and surface tension.
Event Details
Date/Time:
Abstract
It has long been known that weakly nonlinear field theories can have a late-time stationary state that is not the thermal state, but a wave turbulent state (the Kolmogorov-Zakharov state) with a far-from-equilibrium cascade of energy. We go beyond the existence of the wave turbulent state, studying fluctuations about the wave turbulent state. Specifically, we take a classical field theory with an arbitrary quartic interaction and add dissipation and Gaussian-random forcing. Employing the path integral relation between stochastic classical field theories and quantum field theories, we give a prescription, in terms of Feynman diagrams, for computing correlation functions in this system. We explicitly compute the two-point and four-point functions of the field to next-to-leading order in the coupling. Through an appropriate choice of forcing and dissipation, these correspond to correlation functions in the wave turbulent state. As a check, we reproduce the next-to-leading order term in the kinetic equation. The correlation functions and corrections to the KZ state that we compute should, in principle, be experimentally measurable quantities.
Event Details
Date/Time:
Abstract
Tensegrities are mechanical structures that include cable-like elements that are strong and lightweight relative to rigid rods yet support only extensile stress. From suspension bridges to the musculoskeletal system to individual biological cells, humanity makes excellent use of tensegrities, yet the sharply nonlinear response of cables presents serious challenges to analytical theory. Here we consider large tensegrity structures with randomly placed cables (and struts) overlaid on a regular rigid backbone whose corresponding system of inequalities is reduced via analytic theory to an exact graph theory. We identify a novel coordination number that controls two rigidity percolation transitions: one in which global interactions between cables first support external loads and one in which the structure becomes fully rigid. We show that even the addition of a few cables strongly modifies conventional rigidity percolation, both by modifying the sharpness of the transition and by introducing avalanche effects in which a single constraint can eliminate multiple floppy modes.
For recordings of seminars in this series, see https://smartech.gatech.edu/xmlui/handle/1853/63906
Event Details
Date/Time:
Abstract: Penning ion traps are useful experimental platforms for quantum simulation, mass spectrometry, precision metrology, and molecular ion spectroscopy. The GTRI Quantum Systems Division (QSD) has developed a compact, permanent-magnet-based Penning trap that is compatible with cold ion experiments, and we have recently updated our compact trap design with printed-circuit-board electrodes and improved trap magnetic field stability. We describe some near-term applications of this system including compact atomic clocks with dual-species, three-dimensional (3D) ion crystals and demonstration of the quantum approximate optimization algorithm (QAOA) with 2D ion arrays. We also highlight other notable results from the QSD in the field of ion trap quantum information.
Bio: Dr. Brian Sawyer is the Chief Engineer of the Quantum Systems Division at GTRI. He received his Ph.D. in the group of Dr. Jun Ye at JILA/University of Colorado in 2010, where he demonstrated Stark deceleration and magnetic trapping of cold OH molecular beams and performed the first cold collision experiments between OH and ND3 molecules. He subsequently worked in the group of Dr. David J. Wineland at the National Institute of Standards and Technology (NIST) as a National Research Council postdoctoral fellow, where he performed the first quantum simulation experiments using two-dimensional ion arrays in a conventional Penning trap. He now leads two experimental efforts at GTRI developing compact Penning traps for portable atomic clock and quantum simulation applications.
Event Details
Date/Time:
Event Details
Date/Time:
Abstract: We present experiments on the effects of laminar flows on the motion of swimming microbes and on the spreading of the excitable Belousov-Zhabotinsky chemical reaction. A universal theoretical framework of active mixing predicts invariant manifolds -- “burning invariant manifolds” (BIMs) for front propagation and “swimming invariant manifolds” (SwIMs) for self-propelled tracers -- that act as one-way barriers for both of these systems. In fact, the problem of front propagation is a special case of the more general, active mixing theory. We present results from several experiments: (a) BIMs blocking reaction fronts in a range of 2-D and 3-D vortex-dominated flows; (b) SwIMs blocking motion of swimming bacteria and eukaryotic microbes in a microfluidic hyperbolic flow in a cross channel; and (c) on-going experiments about the behavior of swimming microbes in vortex flows.
Bio: Prof. Solomon is a professor in the Department of Physics & Astronomy at Bucknell University in central Pennsylvania. He and his undergraduate research students study chaotic fluid mixing and the effects of fluid mixing on dynamical processes occurring in the flows. Their experiments at Bucknell have been funded by 7 grants from the National Science Foundation and Research Corporation, and have resulted in 30 publications. The research program was recognized by the American Physical Society in 2005 for outstanding research by an undergraduate and in 2014 for outstanding research by a faculty member at an undergraduate institution.
Event Details
Date/Time:
Abstract
We propose a new 'active particle' system in which the particles are in fact polymer-like: the Tubifex tubifex or 'sludge' worm. I will discuss three recent experiments that highlight the richness of this active system. In the first experiment, we perform classical rheology experiments on this entangled polymer-like system. We find that the rheology is qualitatively similar to that of usual polymers, but, quantitatively, (i) shear thinning is reduced by activity, (ii) the characteristic shear rate for the onset of shear-thinning is given by the time scale of the activity, and (iii) the low shear viscosity as a function of concentration shows a very different scaling from that of regular polymers. The level of activity can be controlled by changing the temperature but also by adding small amounts of alcohol to make the worms temporarily inactive.
In a second experiment, we disperse the worm in a quasi-2D aquarium and observe their spontaneous aggregation to compact, highly entangled blobs; a process similar to polymer phase separation, and for which we observe power-law growth kinetics. We find that the phase separation of active polymer-like worms does not occur through Ostwald ripening, but through active motion and coalescence of the phase domains. Interestingly, the growth mechanism differs from conventional growth by droplet coalescence: the diffusion constant characterizing the random motion of a worm blob is independent of its size, a phenomenon that can be explained from the fact that the active random motion arises only from the worms at the surface of the blob. This leads to a fundamentally different phase-separation mechanism, that may be unique to active polymers. Finally, in the remaining time, I will briefly show that we can efficiently separate by size and activity these living polymers using hydrodynamic chromatography technics.
Bio
Antoine Deblais is an assistant professor at the University of Amsterdam since 2021. Before that, he spent two years as a Postdoctoral researcher at the University of Amsterdam (UvA), Netherlands(2017-2019). He worked on many problems involving flows and instabilities of complex fluids in different situations. With the obtention of an individual Marie-Curie Fellowship, he pursued his independent research between Unilever research centre in Wageningen (Netherlands) and UvA on the relationship between rheology of complex fluids and mouthfeel. He completed his PhD at the University of Bordeaux in France in 2016. His research interests span on the flow of complex fluids, capillarity, and active matter.
Event Details
Date/Time:
Pages
