Contact Information
- jg42@gatech.edu
- Phone
- (404) 894-4029 x894
- Location
- Howey N106
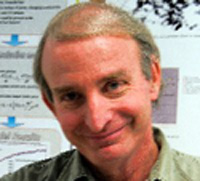
James Gole
Professor Emeritus
Research
Physical phenomena which fall at the interface of chemical and condensed matter physics and material science
Professor Gole's group is concerned with the study of physical phenomena which fall at the interface of chemical and condensed matter physics and material science. It has become evident that the enhanced activity of nanoscale structures can have a profound affect on the development and modification of MEM/NEMS devices and sensing/tagging/microreactor/photovoltaic technology, providing new inroads to create hybrid devices with greatly enhanced sensitivity and selectivity. The understanding of the physical phenomena which guide these processes falls at the interface of Chemical and Condensed Matter Physics. Within this framework, our laboratory has been concerned with (1) the action of nanostructures as they are introduced to nonporous/microporous interfaces and their subsequent ability to enhance interaction and thus to promote an increased sensitivity and/or a more efficient conversion and transduction at these interfaces, (2) the development of new, high yield, nanoscale, exclusive processes forming the novel interactive nanostructures necessary for this effective interface modification, and (3) the development of complementary "active" micro/nano filters. In developing these interfaces, we seek to identify the micro-nanoscale materials phenomenon that form the framework for new interface transformations, photocatalysis-based microreactors, photovoltaic devices, and micro-and nanobattery configurations.
A substantial effort is now channeled into the study of porous (primarily silicon) structures (PS) where the first examples of surface based photoluminescence (PL) induced metallization for electrical contact has been demonstrated. Contacts of extremely low resistance, formed using PL induced metallization techniques are now being used to develop highly sensitive chemical sensors and exploit electroluminescence to develop photocatalysis-based microreactor configurations. In conjunction with select nanostructure deposition techniques, solar pumped chemical and biosensors are under development. Chemical and biosensors are being greatly improved with the application not only of nanostructured and photocalalytic surface coatingo but also through the application of fast Fourier transform techniques and stereolithography. Further, in concert with nanotechnology, we are developing new photocatalytic microreactor configurations.
Semiconductor nanostructures, nanoagglomerates, and nanowires have attracted considerable attention because of their potential applications in mesoscopic research, the development of nanodevices and the potential use of large surface area structures for catalysis. In conjunction with efforts in porous silicon (PS), our research is also focused on the formation and characterization of silicon, silica, tin oxide, titania, and zirconia-based nanostructures, the effects of main group (N,F) seeding and highly concentrated metal atom doping on these uniquely active structures.
We have applied the techniques of high temperature synthesis to generate virtually defect free SiO2 sheathed crystalline silicon nanowires and gram quantities of silica (SiO2) nanospheres which can be agglomerated to wire-like configurations impregnated with crystalline silicon nanoclusters. This nanoagglomeration was extended to generate crystalline silicon impregnated SiO2 nanowire "bundles" and "brush-like" arrays and the first silica nanotubes. The SiO2 nano-sphere powders, which themselves have been found to catalyze hydroxylation reactions, have also been used to form large surface area selective Cu/SiO2 and transition metal-based catalysts.
PS metallization techniques have been used to fabricate ferromagnetic nickel, silver, and iron-coated silica cored nanospheres. Concomitantly in connection with porous silicon based microreactor development, the Gole group has formulated new extrememly efficient techniques to form TiO2-xNx nanophotocatalysts that have the important property of absorbing light throughout the visible spectral region, therefore, more efficiently using energy from the solar spectrum. The success of this work results from the enhanced of interaction characteristic of quantum dots and the improvement in synthesis efficiency obtained upon traversal to the nanometer regime. This unique activity at the nanoscale is the centerpiece of new experiements in our laboratory which have now been extended to the nitritdation of additional catalytic metal oxides and molecular sieves including faujasite. Here, we find significant improvements in catalytic activity associated with profound changes in molecular electronic structure.
Novel nano photocatalysts are now being used in conjunction with porous silicon (PS) to develop highly effcient micro/nanoreactors and solar pumped sensor configurations. Most recently, this work with porous nanostructures has been extended to study the insertion of magnetic dopant ions used to replace titanium in an anatase TiO2 lattice in order to produce dilute magnetic semiconductors. With these appproaches, for example, an extremely rapid route for the room temperature phase transition of anatase to rutile TiO2 has been discovered.The conversion which typically requires temperatures in excess of 850 degrees celcius for 12 hours is now reduced to one minute at room temperature. Finally, with a nanoscale combined nitrogen doping - high spin transition metal ion seeding, a means to grealy enhance the infrared signatures of minor contaminants at the interface of a doped oxynitride surface has been developed.
Recently we have also successfully formed zirconium oxide nanoshells and hollow nanospheres with potential applications in fuel cell, hydrogen storage, and thermoelectric power generation.
Nanostructure Related Research-Short Summary
This group is concerned with the synthesis and characterization of nanostructures, and the nature of their incorporation into porous media. Porous interfaces (primarily silicon) are transformed within the framework of nanotechnology using a variety of nanostructures to create highly efficient sensors, interactive support surfaces, active battery electrodes, and novel nanophotocatalytic microreactor arrays. Silica (nanospheres, nanowire arrays, and nanotubes), tin oxide (ground state and metastable nanowires, ribbons, and nanotubes), and doped titania and zirconia-based nanostructures are used to create highly active frameworks. Novel, "active" silica nanosphere powders whose unique oxidation states create uniquely active support surfaces, within themselves have been found to catalyze hydroxylation reactions. Further, they have also been used to form large surface area selective Cu/SiO2 and transition metal ion catalysts. New nanoscale exclusive techniques have been developed for the rapid formation of TiO2-xNx nanophotocatalysts operative in the visible wavelength region to be used in conjunction with porous silicon hybrid nanopore coated micropores to form novel and efficient solar pumped sensors and microreactors. Novel ZrOx nanoshells and hollow nanospherical forms have been synthesized and their applications to fuel cell, hydrogen storage, and thermoelectric power generation are under study. This work is now being extended to several metal oxides and to the faujasites.
Nanostructure Research in the Gole Laboratory (see publications following)
Synthesis, Characterization, and Incorporation of Nanostructures
Enhanced Activity, Reactivity, Catalysis, and Phase Transformation at the Nanoscale
Unique Silica Nanopheres, Nanotubes, and Nanofiber Arrays
We have used a modified-flow tube furance configuration carefully calibrated for temperature, temperature gradients, entrainment gas flow rate, and total pressure, and variable Si/SiO2 mixtures to generate uniquely active silica (SiOx) nanospheres which not only provide a support from which to generate enhanced catalytic activity but also display an unexpected reactivity. These structures can also be agglomerated to wire-like configurations subsequently providing a means to grow silica nanotubes.
Fig 1. TEM of virtually "monodisperse" SiO2 nanospheres, 30 nm in diameter, synthesized by gas phase condensation from Si/SiO2.
Fig 2
Characteristics of Silica Nanostructures*
- Characterized by New and Unique Silicon Oxidation States
- Form Improved Selective Support Surfaces-Improved Selective Catalyst Supports
- Enhanced Activity and Reactivity Relative to Fumed Silica (Cab-O-Sil) the commonly used nanostructured silica
- Average Silicon Oxidation State of (+3) vs. Fumed Silica at (+1), a new and startling result
- Syntheses are Environmentally Benign vs. Fumed Silica
- Applications in Microreactors
*See Gole posters (2)
Ready Phase Transformation at the Nanoscale
SnOx nanostructures* can be synthesized which, at pressures of a few hundred Torr, display a phase coexistence between rutile and orthorhombic crystal structures normally observed at pressures in excess of 150 kbar in the bulk. These coexisting phases are indicated in the figure below. Similar ready phase transformations can be catalyzed in TiO2, ZrO2, and several other metal oxide nanocrystals. These metastable phases may provide enhanced sensitivity and activity as they interact with support surfaces, including silica, faujasites, and gamma alumina structures.
* See also Gole posters (1)
From Nanostructures to Nanostructure Modified Micro/Nanostructured Porous Silicon Sensors and Micro/Nano Reactors
Porous Silicon Interfaces
Interfaces and their modification will continue to play increasing roles as they are transformed within the framework of nanotechnology to develop important devices based in sensors, interactive support surfaces, and nanophotocatalysis. Variably porous silicon surfaces that are either functionalized and/or modified via the select deposition of uniquely generated quantum dots to greatly improve the sensing of select reagents or, alternatively, catalytic activity. Porous silicon (PS) formed from the etch of a silicon wafer is characterized by an extensive visible photoluminescence (PL) which when excited by uv radiation displays a time dependent behavior resulting primarily from surface-based oxidation processes. The first time dependent PL histograms recorded in our laboratory for PS reflect the summation of light emission over a number of sites on the PS surface as they demonstrate the evolution of transitions in surface bound emitters extending and transforming from the blue-green to the orange-red region of the spectrum within a time scale extending from 1.5 up to 70µs following the uv excitation. The development, enhancement, and evolution of this PL can be significantly influenced through the introduction of a variety of surface treatments and through the introduction of select nanostructures.
Porous Silicon Sensors
The development of devices based on the porous silicon PL, while a focus of considerable effort, has been slowed by (1) the inability to enhance and stabilize this PL for long periods and (2) the inability to obtain a low resistance contact to the PS surface as typical resistances are of the order kΩ-MΩ. In overcoming this primary limitation in the application of PS, we have demonstrated that the PL from nanoporous or hybrid macroporous-nanoporous PS structures can be greatly enhanced and stabilized. More recently, based on this PL enhancement, we have developed a technique for the selective patterned metallization of a porous silicon (PS) surface using the photoluminescent surface as a reducing agent. We take advantage of the intimate environment associated with a controlled photoluminescence-based metallic ion reduction from electroless solution. The basis of this method lies in the use of the stabilized "long-lived" PS luminescence to enhance reduction at the PS surface. The formation of surface bound electronically excited "centers", whose interaction and reaction capability greatly exceeds that for an unexcited surface, provides a greatly enhanced and controllable reduction is used to achieve contact resistances in the 20-100 Ω range.
Over the past several years, our group have shown that PS sensors represent a robust technology for a wide range of conductometric detection applications. To date, they have developed 1-7 rapidly responding, reversible, sensitive, and selective PS sensors which operate at room temperature and can be readily rejuvenated. With a highly efficient electrical contact formed to a nanostructure modified nanopore covered microporous channel array and (2) selective nanostructure coating to create an environment for physisorption, these sensors are capable of operation in a high temperature flue gas environment. The technology implemented on “phase matched” PS nanopores facilitates the application of nanostructured metals, metal oxides, and catalytic coatings, providing for notably higher sensitivities and selectivity. Materials which include electroless gold (AuxO), tin (SnO2), copper (CuxO), nickel (NiO), and nanoalumina provide for the detection of gases including NH3, PH3, CO, NO, H2S, and SO2 in an array-based format at the intermediate or lower ppb level. The value of this conductometric sensor technology results from a combination of (1) its sensitivity and short recovery time, (2) its operation at room temperature as well as at a single, readily accessible, temperature with an insensitivity to temperature drift, (3) its potential operation in a heat-sunk configuration allowing operation to a surface of 80°C even in highly elevated temperature environments (in sharp contrast to metal oxide sensors), (4) its ease of coating with a diversity of clearly mapped gas-selective materials for form sensor arrays, (5) its low cost of fabrication and operation, (6) its low cost and ease of rejuvenation following contamination, and (7) its ability to rapidly assess false positive signals using FFT techniques, operating the sensor in a pulsed gas mode.
We have formulated a general approach to facilitate significant and predictable changes in sensor surface sensitivity for a variety of gases based on a complementary theory to the well known concept of hard and soft acid and base interactions by Pearson and others.8-12 Nanostructured sensor surfaces for conductometric array-based devices are constructed from a finders table by first establishing whether the gas to be detected classifies as a hard or soft acid or base and subsequently developing nanostructured coatings which encompass as wide a range of acidity and basicity as possible. In addition, the correlation with trends in the proton affinity (PA), essentially the gas phase basicity, of the gases of interest is considered.13 A maximum response (impedance change) is to be expected from combinations of hard acids with soft bases and vice versa, however other factors, including steric effects, and polarization14 can be used to advantage.
Exemplary are extensive studies involving the sensing of PH3, NH3, and NO on several nanostructure modified PS surfaces summarized in Table 1. The results of which we have obtained for phosphine are exemplified in Figure 5 for an AuxO nanostructure coated micro/nanoporous PS surface. Figure 5 demonstrates that the impedance change for an AuxO nanostructure coated surface is close to five times that of the untreated surface. Table 1 also includes additional new data obtained for NO15 with electroless copper, nickel, and gold treatments for Cu+1, +2 and Ni+2 oxides, and Au+1,0 clustered oxide nanostructured coatings.
Fig 5. Improved PH3 response to an electroless AuxO coating. Here, 1,2,3,4, and 5 ppm of PH3 is pulsed onto the sensor surface every 300s. For the first 1800s an N2 purge is applied for resistance stabilization.
In addition, data obtained for CO, a weak base, demonstrates a significant response increase upon exposure of this gas to an SnOx (hard acid) nanostructure coated surface whereas the data obtained for H2S (intermediate base) indicates a significant increase in response for an AuxO nanostructured oxide (weak acid) coated surface.
Table 1. ∆R(coating)/∆R(uncoated) values are shown for PH3, NO, and NH3 impedance changes. Comparison is to an uncoated PS sensor.The nanostructured coatings deposited to the PS surface are indicated in the Table.Base resistance of the sensors used in these experiments varies from 300 to 500 Ω. Further data points for CO, PH3, and H2S are discussed in the text.
Figures 5-7 and Table 1 suggest that the proper combination of nanocoating techniques can be used to produce combinations of array-based devices of varying sensitivity to a variety of gases and that this matrix of array responses can be used in tandem to selectivity analyze gas mixtures. For example, an array of an untreated SnOx nanocoated, and gold clustered oxide coated sensors could be used to sensitively test for the presence and relative concentrations of ammonia and nitric oxide. The nanostructured SnOx/AuxO sensor coatings provide a basis for developing a sensitive room temperature detector that could be installed as a simple sensor system for asthmatics.16
Fig. 6. Comparison of response for sensors that are untreated, treated with electroless gold, or treated with electroless tin, and tested with 30 repeat pulses of 20 ppm NOx, NH3, or CO. The average impedance change is given.
Fig. 7. Significantly improved sensitivity to ammonia vs. the average impedance changes given in Fig. 6. The experiments are run in an identical configuration.
The Gole group has also developed the ability to rejuvenate poisoned sensors. This is adequately demonstrated in Figure 8.
Fig. 8. Response to NH3 after a sensor is subjected to a rejuvenation process.
References
- J.L. Gole, “A General Approach to Creating Selective Metal Coatings at a Nano/microporous Porous Silicon Interface for Sensor Applications,” Continuation in Part Patent Applied For.
- S.E. Lewis, J.R. DeBoer, J.L. Gole, and P.J. Hesketh, “Sensitive, selective, and analytical improvements to a porous silicon gas sensor,” Sensors and Actuators B 110:54 (2005).
- J.L. Gole, S.E..Lewis, and J.R. DeBoer, “Pulsed System Frequency Analysis for Device Characterization and Experimental Design,” Sensors and Actuators, in press.
- J.L. Gole, S. Lewis, and S. Lee, “Nanostructures and Porous Silicon: Activity at Interfaces in Sensors and Photocatalytic Reactors,” Phys. Stat. Sol., in press.
- J.L. Gole, S.E. Lewis, A. Fedorov, and S. Prokes, “Nanostructures and Porous Silicon: Activity and Phase Transformation in Sensors and Photocatalytic Reactors,” Physical Chemistry Interfaces and Nanomaterials IV, SPIE Proceedings 5929-08 (1-12) (2005).
- R.G. Pearson, “Absolute Electronegativity and Hardness: Application to Inorganic Chemistry,” Inorg. Chem. 734-740 (1988).
- See for example:
- Pearson RG. Hard and soft acids and bases—the evolution of a chemical concept. Coordin Chem Rev 1990; 100:403-25. See also
- Pearson RG. Hard and soft acids and bases. J Am Chem Soc 1963; 85:3533-3539.
- Pearson RG Chemical hardness. 1997; Weinheim:John Wiley VCH.
- Pearson RG. Chemical hardness and density functional theory. J Chem Sci 2005; 117(5):369-377.
- Pearson RG. Absolute electronegativity and hardness: application to inorganic chemistry. Inorg Chem 1988; 27:734-740.
- Parr RG, Pearson RG. Absolute hardness: companion parameter to absolute electronegativity. J Am Chem Soc 1983; 105:7512-7516.
- (a) Parr RG, Yang W. Density functional theory of atoms and molecules. 1989; Oxford University Press: New York. (b) Geerlings P, De Proft F, LangenaekerW. Chem Rev 2003; 103:1793.
- Cohen MH, Wasserman A., J Phys Chem 2007; 111:2229-2242.
- Zhan CG, Nichols JA, Dixon DA. Ionization potential, electron affinity, electronegativity, hardness, and electron excitation energy: molecular properties from density functional theory orbital energies. J Phys Chem A 2003; 107(20):4184-4195.
- Dixon D.A. Private communication. See JL Gole and DA Dixon to be published.
- P. Politzer, “Relationship between the charge capacity and the hardness of neutral atoms in group” J. Chem. Phys. 86:1072 (1987).
- Ozdemir S, Gole JL. Work in preparation.
- See
- Lewis S, DeBoer J, Gole JL, Hesketh P. Sensitive, selective, and analytical improvements to a porous silicon gas sensor. Sens Actuators B: Chem 110;54-65 (2005).
- Gole JL, Lewis S, Lee S. Nanostructures and porous silicon: activity at interfaces in sensors and photocatalytic reactors. Phys Stat Sol A 2007; 204: 1417-22 and references therein.
The selectivity and sensitivity of this PS sensor is being improved and enhanced by introducing further quantum dot structures into the pores of PS. These might include (Fig. 7) nitrided silica or titania nanostructures (SiO2-xNx, TiO2-xNx). When introducing nitrogen doped visible light absorbing TiO2-xNx photocatalysts into the pores of the PS framework, we create the possibility for solar or room light pumped sensors based on this oxynitride catalyst. Further, within this interfacial framework, it is also possible to conceive the development of a conceptually novel photocatalytic reactor.
Porous Silicon/QD Microreactors
A porous silicon (PS) structure whose micron-size open pores are decorated by clusters of nitrogen doped silica or titania oxynitride nanospheres (Fig. 7) by themselves, or impregnated with a noble metal, forming appropriate size photocatalyst quantum dots (QD), constitutes a conceptually novel heterogeneous photocatalytic microreactor with the potential for considerably improved performance. Its success might be based on three major factors. First, micro-PS can provide a uniform and highly effective (i.e., tightly localized) illumination of the photocatalyst using the VIS light generated through PS electroluminescence or photoluminescence. Second, PS, especially in the form of a hybrid macroporous-nanoporous structure, can provide an extremely large specific (i.e., per unit volume) surface area owing to its highly porous structure and to the additional surface area made available via attached catalyst-based nanostructures. Third, photocatalysts in the form of nanoparticles have been shown to often possess superior catalytic properties as compared to the conventional bulk catalyst by virtue of the electronic modification of the catalyst through size quantization. Entailed in this concept is the requirement that the photocatalyst quantum dots absorb and convert visible light. Here a nanoscale exclusive synthesis of visible light absorbing TiO2-xNx photocatalysts is essential.
Fig 9. Principle: Use of Nanostructured Porous Silicon Decorated with TiO2-xNx Nanoparticles as a Conceptually New Design for a Portable Photocatalytic Device for the Energy Efficient Oxidation of Pathogenic Bacteria and Viruses.
Forming Visible Light Absorbing TiO2-xNx Photocatalysts
There has been a long, continued, interest in TiO2 based photocatalysis because of the relatively high reactivity and chemical stability of the oxide under uv light excitation (l <387 nm) where this energy exceeds the bandgap of anatase (3.2 eV) crystalline n-TiO2. However, anatase TiO2 is a poor absorber in the visible region. The cost and accessibility of uv photons make it desirable to develop photocatalysts which are highly reactive under visible light excitation utilizing the solar spectrum or even interior room lighting.
We have produced visible light absorbing TiO2-xNx nano-particles in a controlled size range (3-10 nm) in seconds at room temperature employing the nanoscale exclusive direct nitridation of TiO2 nanocolloids using alkyl ammonium compounds. These titanium oxynitrides demonstrate a high quantum yield (> 0.2) for reductive photocatalysis with methylene blue and for ethylene oxidation on a surface where the quantum yield in the visible is easily comparable to the quantum yield under UV irradiation. An advantage of the nanoscale doping procedure is that it produces slurries of the nitrided particles that form viscous solutions whose viscosity can be readily adjusted. This is of practical advantage as one places the agglomerates on a surface (for example a quartz reactor tube) to create a film of photocatalytically active sites. A further advantage of these colloidal solutions is the unique property by which they can incorporate and uniformly disperse metal compounds and the manner in which these metal compounds can be introduced in concert with the nitriding process. In many instances the introduction of the metal compounds produces a solution which after some controlled period of time can be transformed to a gel. This process can be accelerated by minimal heating (e.g., ~50oC).
As optical reflectance spectra for neat and nitrided TiO2 nanocolloids are compared with the corresponding spectra for Degussa TiO2 at an average size of 30 nm, we observe (1) the effects of quantum confinement (size quantization) and (2) a considerable red shift of the nitrided nanocolloid. The spectrum for Degussa P25 onsetting sharply at l~ 380 nm, the reflectance spectrum for the nitrided (TiO2-xNx) (>3, < 10) nm nanoparticles, rising sharply at 450 nm, and the corresponding spectrum for the nitrided (TiO2-xNx) partially agglomerated nanoparticles rising sharply at 550 nm. This characterization by reflectance spectroscopy emphasizes that we can control the absorption of light through the nitriding protocol and subsequent processing steps. Note also that these processes produce a tuning through the visible region. Further, we have introduced Pd into a nitriding amine-TiO2 mixture. The corresponding reflectance spectrum, obtained as palladium incorporation produces the impregnation of the TiO2-xNx structure with reduced Pd nanocrystallites and the apparent transformation of some of the TiO2-xNx anatase structure, displays an even broader response extending to considerably longer wavelength, albeit at a notably lower light absorption efficiency as a function of wavelength.
Fig 10. Reflectance measurements on different TiO2 samples showing the red-shift upon nitrogen-doping in the absorption of the nanocrystals, and the additional red-shift with increasing cluster size.
Fig 11. Visual comparison of a). TiO2 nanoparticles, b). Degussa P25 TiO2 powder, c). Nitrided TiO2-xNx nanopowder, d). Degussa P25 TiO2 powder nitrided with Triethylamine; 1B) UV-visible reflectance spectra of a) TiO2 nanoparticles, b) Degussa P25 TiO2 powder, c) nitrided Degussa P25 TiO2-xNx powder, and d) nitrided TiO2-xNx nanopowder.
A further comparison is in order. If we compare (Fig. 11) the reflectance spectra for neat and nitrided TiO2 nanocolloid and Degussa P25 TiO226 the latter of which is nitrided over a time period of days. The efficiency of the nitriding process for Degussa P25 is minimal compared to that of the colloid and the corresponding reflectance spectrum is notably more complex than that for the nitrided nanocolloid. Note also that the nanocolloid reflectance spectrum is blue shifted relative to the Degussa sample, consistent with a smaller size particle distribution. It is, however, striking that the nitrided nanocolloid displays a reflectance spectrum notably red shifted from that of the Degussa sample. A Debye-Scherrer analysis of the XRD patterns of Degussa P25 and a nitrided and partially agglomerated TiO2 colloid (550 nm feature, Figure 10) shows a broadened pattern for the nanocolloid that suggests a size close to 10 nm. In contrast to this nanoparticle activity, no measurable reaction or heat release is observed as either distinct rutile or anatase TiO2 micropowders are treated.
Fig 12. Comparison of the photocatalytic decomposition of Methylene Blue after a) 390 nm laser excitation and b) 540 nm monitored at 650 nm, catalyzed by undoped TiO2 (blank test, open diamonds) and Nitrogen-doped TiO2 nanocrystals. The insert in 4a shows the photodegradation of methylene blue in water at neutral pH.
Photocatalytic Activity
Methylene blue decolorization in water (either aerobic or anerobic) is a probe reaction often cited to establish the photocatalytic activity of a novel photocatalyst. We have observed methylene blue decolorized under aerobic conditions for irradiation at 390 and 540 nm at a pH of 7 and for various neat and Pd-seeded-Ti-O-N catalysts. Photocatalytic activity has been evaluated at 390 and 540 nm. The data for the nitrided samples as well as the palladium treated sample referred to above are consistent with a notably enhanced activity for the TiO2-xNx constituencies at 390nm whereas the undoped TiO2 nanoparticles showed minimal activity under visible light irradiation. The significant decrease in the optical density for methylene blue in the presence of the synthesized TiO2-xNx photocatalyst, upon visible light excitation, signals a conversion process that displays a notable activity relative to a blank and TiO2 sample. Notable decreases in optical density are observed at 540 nm, but differences in activity are muted in this less sensitive absorption region. In contrast, at wavelengths below 350 nm, the activity of both the TiO2 and nitrided samples is comparable. Thus nitrided TiO2-xNx samples that can be generated in several seconds at room temperature are catalytically active at considerably longer wavelength than TiO2. While these results are very encouraging for the dispersed colloids in solution, the gas phase oxidation of an olefin using dioxygen is a more demanding test for the application of this science to the grand.
Visible Light Active Photocatalytic Films
Films constructed from colloidal solutions of TiO2-xNx and Pd-TiO2-xNx on a quartz surface are photocatalytically active for the total oxidation of ethylene to carbon dioxide under UV and incandescent light illumination at room temperature as measured in a stop-flow microreactor. Unlike the untreated TiO2 photocatalyst, films composed of nitrided TiO2 colloid centers display a significant photocatalytic activity under illumination by the primarily visible light generated from the incandescent lamp at a much lower photon flux. The visible response is therefore greater than that for pure UV excitation. This result demonstrates unambiguously that the nitriding procedures we have employed can produce surface based doped titania photocatalyst centers which are catalytically active within a broad range of optical excitation well into the visible range. These results provide strong evidence that novel materials which can be synthesized at room temperature can be used to coat silicon-based surfaces which, under visible light illumination, correspond to strongly oxidizing photocatalytic centers that formed a low temperature, selective, surface oxidation catalyst. We can generate uniquely optimized photocatalytic films by modifying the nature of those centers that produce the photocatalytic activity. The use of these films to produce quantum dot coated PS pores will represent a major future effort in our laboratory.
Fig 13a
CO2 formation measuring the photocatalytic oxidation of ethylene in a stop-flow reactor loaded with TiO2 nanostructures under UV (365 nm), incandescent lamp (primarily in the visible range), and no illumination at room temperature.
Fig 13b
CO2 formation measuring the photocatalytic oxidation of ethylene in a stop-flow reactor loaded with Pd-TiO2-xNx nanostructures under UV lamp (365 nm), incandescent lamp (primarily in the visible range), and no illumination at room temperature (photon flux in visible ~1/5 flux in UV)
Further Applications of Doped and Metal/Seeded TiO2
There are other potential applications of the TiO2 based doping/seeding at the nanoscale. In view of the unique ability of these nanocolloid solutions to incorporate transition metal compounds, we have considered the potential photovoltaic applications resulting from the incorporation of readily ionized metals including transition metals Co, Fe, Ni, V, Pt and silver into the TiO2 and TiO2-xNx lattice. Here, initial studies with silver have revealed a very intriguing chemistry that might be generalized using a number of mixed metal combinations. While silver crystallites can be incorporated and dispersed into TiO2 or its nitrided analog, TiO2-xNx, Figure 14 demonstrates assembled silver oxide based arrays. These are formed as this crystalline material is interspersed within colloidal TiO2 as the entire assembly takes on an assembled needle-like shape. These needle-like arrays are formed upon introduction of a zinc metal probe into a silver nitrate impregnated lattice. We suggest that the self-assembly may be induced through the formation of a zinc hydroxide hydrogel. Experiments are currently underway to verify this suggestion. In addition, the nature of these metal seeding experiments with silver suggests the possibility of creating an environment which can be used to greatly amplify a Surface Enhanced Raman signal to detect the vibrational modes of single molecules trapped on a surface. Here, we refer to the recently reported extraordinarily high SERS enhancement factor used to achieve the detection of the vibrational spectra of single molecules on a surface. The presence of a confined metal/metal clustered oxide framework in concert with a photocatalytic source enhances this possibility.
(a) TEM micrograph of the probe induced assembly of silver oxide crystallites on TiO2. (b) Closer view of silver oxide crystallites assembled on TiO2. (c) Closeup of silver oxide crystallites on which Moire Patterns are visible.
Further metal seeding experiments can be envisioned. The porous nature of the TiO2 and TiO2-xNx nanocolloids facilitates their ability to incorporate metals into their anatase framework. With a significant Ni or Co loading, far exceeding the typical 0-5% levels currently attained, it might well be possible to create dilute magnetic semiconductors. We have already produced uniformly loaded Co and Ni seeded TiO2 and TiO2-xNx constituencies. In fact, the oxidation state of Co is found to change from +2 to +3 depending on whether the metal is seeded into TiO2 or TiO2-xNx. These systems are currently being studied to determine their Curie temperature and the nature of their magnetic domains.
Solar Pumped Sensors
It has become desireable to develop highly stable and inexpensive pollutant mediating photocatalysts which have significant activity under visible light excitation utilizing the solar spectrum or even interior room lighting. Once developed, however, the utilization of visible light absorbing photocatalytic materials can offer the potential for the significant improvement of sensor suites. Here solar and/or interior room lighting absorbed by a photocatalyst is used to produce electron-hole pairs, either of which might be used to enhance the sensing process by modifying the interfacial depletion layers of the chemically sensitive metal oxide layer. We create the basis for a solar pumped sensor.
Synthesis of Zirconium Oxide Nanoshells and hollow Nanospheres
In a series of novel experiments we have successfully synthesized Zirconium Oxide Nanoshells and hollow Nanosphere configurations. The products of this unusual synthesis may have a number of exciting applications. In addition to their potential use in forming high k dialectic layers, the hollow nanospheres may provide new routes for fuel cell and hydrogen storage media. Further the photoluminescence (PL) properties of this wide band gap oxide may find application for the development of optical memory systems. Here, it is especially important to note that the hemispherical nature of the ZrO2 nanospheres (below) can facilitate an enhanced PL versus that of alternate ZrO2 nanoparticles. Finally, we know that the nature of the hollow ZrO2nanopheres is such that the combination of their Seebeck coefficients, electrical, and thermal conductivity suggest that they will have a high figure of merit for thermoelectric power generation. Measurements of the electrical and thermal conductivity are now underway on these systems.
A Blossoming Group of ZrO2 Nanoshells
ZrO2 Nanoshells
Fractal Structure Associated with the Combination of "Hollow" ZrO2 Nanospheres. These nanospheres and their Metallized Counterparts are being tested for their Ability to Uptake and Store Hydrogen
Papers
A. Nanoscience, Nanotechnology, Nanocatalysis
- Nanostructures-Characterization and Structural Properties
- Nanostructure Directed Physisorption vs. Chemisorption at Semiconductor Interfaces: The Inverse of the Hard-Soft Acid-Base (HSAB) Concept” James L. Gole and Serdar Ozdemir, Chem. Phys. Chem., in press.
- "Visible-light-driven Reversible and Switchable Hydrophobic to Hydrophilic Surfaces: Correlation with Photocatalysis", Junwei Wang, James L. Gole, and Clemens Burda, Nanoscale, submitted.
- “Synthesis of Nanoscale Silicon Oxide Oxidation State Distributions: The Transformation for Hydrophilicity to Hydrophobicity,” James L. Gole, Mark G. White, William Laminack, A.G. Ogden, Jon Brauer and David Dixon, J. Phys. Chem., submitted.
- “Concentration Dependence of the Iron Ion Doping of Titanium Oxide and its Oxynitride: Charge Transfer, Phase Transformation, and the Analog of Nitrogen Fixation,” James L. Gole, J. Brauer, C. Burda, S.M.Prokes, O.J. Glembocki, and J. Wang Adv. Func. Materials., submitted.
- “Metal Ion Induced Room Temperature Phase Transformation and Stimulated Infrared Spectroscopy on TiO2 – based Surfaces”, James L. Gole, S.M. Prokes, and Mark G. White, Applied Surface Science, 255, 718 (2008).
- “Study of Concentration-dependent Cobalt Ion Doping of TiO2 and TiO2-xNx at the Nanoscale,” James L. Gole, Sharka M. Prokes, Xiaofeng Qiu, Clemens Burda, and Orest J. Glembocki, Nanoscale, in press.
- “Creating Looser and More Basic Zeolite Structures from Direct Low-Temperature Nitridation”, James L. Gole, Jonathan Brauer, Samuel Graham, Jhizang Hu, and D.A. Dixon, Nature Materials, submitted.
- “Hydrolysis of TiCl4: The Initial Steps in the Production of TiO2” T-H. Wang, A.M. Navarrette-Lopez, S. Li, D.A. Dixon and J.L.Gole, J.Phys. Chem.C, in press.
- "Maintaining Particle Size in the Transformation of Anatase to Rutile Titania Nanostructures", Andrew Ogden, Andrei Fedorov, Jong-Il Hong, and James L. Gole, J. Phys. & Chem. of Solids, 69, 2898-2906 (2008).
- "The Surprising Average Oxidation State of Fumed Silica and the Nature of Water Binding to the Silicon Oxides and Hydroxides", James L. Gole, Mark G. White, Tsang-Hsiu Wang, and D.A. Dixon, Molecular Catalysis A, submitted.
- "Diffusion Controlled Self-Assembly and Dendritic Formation in Silver Seeded Anatase Titania Nanospheres", James A. Corno, John Stout, Rusen Wang, and James L. Gole, J. Phys. Chem. C, 112, 5339-5346 (2008).
- Optical and Electronic Properties of Semiconducting Nanostructures for Photocatlytic Hydrogen Generation", A. Ogden, J.L. Gole, and A.G. Fedorov, Journal of Nanoelectronics and Optoelectronics I, invited, 2,269-277 (2007).
- "Development of Porous Silicon-based Active Microfilters", Jenna Campbell, James A. Corno, Nicole Larsen, and James L. Gole, Journal of Electrochemical Society, 155 D128-D132 (2008) - Web. of Science November 2007.
- "Microstructured Nanopore-walled Porous Silicon as an Anode Material for Rechargeable Lithium Batteries", Dae-Geun Kang, James A. Corno, James L. Gole, and Heon-Cheol Shin, Journal of Electrochemical Society, 155, A276-A281 (2008).
- "Evidence for High Spin Transition Metal Ion Induced Infrared Spectral Enhancement", James L. Gole, Sharka M. Prokes, Mark G. White, Tsang-Hsiu Wang, Raluca Cracium, and David A. Dixon, J. Phys. Chem. C, 111, 16871-16877 (2007).
- "Efficient Room Temperature Conversion of Anatase to Rutile TiO2 Induced by High Spin Ion Doping", James L. Gole, Sharka M. Prokes, and Orest J. Glembocki, J. Phys. Chem. C, 1782-1788 (2008).
- "Metal Ion Induced Room Temperature Phase Transformation and Stimulated Infrared Spectrscopy on TiO2- based Surfaces", James L. Gole, S.M. Prokes, and Mark G. White, Applied Surface Science, 255, 718 (2008).
- "Nanostructures and Porous Silicon: Activity at Interfaces in Sensors and Photocatalytic Reactors", J.L. Gole, S. Lewis, and S. Lee, Phys. Stat. Solids, (a) 204, No. 5, 1417-1422 (2007).
- "Optical Properties of In-Situ-Doped and-Undoped Titania Nanocatalysts and Doped Titania Sol-Gel Nanofilms", A. Ferreira da Silva, I. Pepe, J.L. Gole, S.A. Tomas, R. Palomino, C. Persson, R. Ahuja, W.M. de Azevedo, and E.F. da Silva, Jr., Journal of Applied Surface Science, 253, 5365 (2006).
- "Unique Properties of Selectively Formed ZrOx Nanostructures - Light Enhancement from a Metal Oxide", J. L. Gole, S. M. Prokes, J. D. Stout, O. J. Glemboski, and R. Yang, Advanced Materials, 18, 664 (2006).
- "Nanostructures and Porous Silicon: Activity and Phase Transformation in Sensors and Photocatalytic Reactors", J. L. Gole, S. E. Lewis, A. Fedorov, and S. Prokes, in Physical Chemistry of Interfaces and Nanomaterials IV, SPIE Proceedings 5929-08 (1-12), (2005).
- "Nanostructure and Morphology Modified Porous Silicon Sensors", J. L. Gole, S. E. Lewis, in Quantum Sensing and Nanophotonic Devices - Nanostructures in Silicon, SPIE-Proceedings, Volume 5732, 573-583 (2005).
- "Unusual Properties and Reactivity at the Nanoscale", with Clemens Burda, Z. L. Wang, and Mark White, Physics and Chemistry of Solids 66, 546 (2005).
- "Defect Related Optical Behavior in Surface Modified TiO2 Nanostructures", with S. M. Prokes and W. E. Carlos, Advanced Functional Materials, 15, 161-167 (2005).
- "A Suggested Oxidation State Dependence for the Activity of Nanostructures Prepared from Tin/Tin Oxide Mixtures", James L. Gole, Alexei V. Iretaskii, Mark G. White, Amanda Jacob, W. Brent Carter, Sharka M. Prokes, and Ann S. Erickson, Chemistry of Materials, 16, 5473-81 (2004).
- "Defect Formation in Surface Modified TiO2 Nanostructures", S.M. Prokes and J.L. Gole, Nanoparticles and Nanowire Building Blocks – Synthesis, Processing, Characterization and Theory, 818, M5.12 (2004).
- "A Suggested Oxidation State Dependence for the Activity of Nanostructures Prepared from Tin/Tin Oxide Mixtures", James L. Gole, Alexei V. Iretaskii, Mark G. White, Amanda Jacob, W. Brent Carter, Sharka M. Prokes, and Ann S. Erickson, Chemistry of Materials, 16, 5473-81 (2004).
- "Surface Oxidation States in Si/SiO2 Nanostructures Prepared from Si/SiO2 Mixtures", James L. Gole, Brian D. Shinall, Alexi V. Iretskii, Mark G. White, and Ann S. Erickson, Langmuir, 20, 260-62 (2004).
- "Surface Modification and Optical Behavior of TiO2 Nanostructures", with S. M. Prokes, W. E. Carlos, C. She, and T. Lian, MRS Proceedings. "Spatially Resolved Characterization of Locl Phenomena in Materials and Nanostructures", 738, 239 (2003).
- "Silica Based Nanospheres, Nanowires, Nanosubstrates, Nanotubes, and Nanofiber Arrays", J. L. Gole, Z. L. Wang, Z. R. Dai, J. Stout, R. P. Gao, and Mark White, invited paper, Progress in Colloid and Polymer Science 281, 673-685 (2003).
- "Study of the Formation of Ferromagnetic Ni/SiO2 Nanospheres", with S. M. Prokes, W. E. Carlos, Lenward Seals, and Stephen Lewis, Materials Letters 54, 85 (2002).
- "Measuring the Young's Modulus of Solid Nanowires by in-situ TEM", with Z. L. Wang, Z. R. Dai, and R. P. Gao, J. of Electron Spectroscopy 51, 579-583 (2002).
- "Formation of Ni/SiO2 and Ag/SiO2 Nanosphere Composites", with S. M. Prokes, W. E. Carlos, Lenward Seals, and Stephen Lewis, Materials Research Society, Proceedings, Nanoparticulate Materials 704, 343 (2002).
- "Tin Oxide Nanowires, Nanoribbons, and Nanotubes", with Z. R. Dai, Z. L. Wang, and J. D. Stout, J. Phys. Chem. B106, 1274-9 (2001).
- "SnOx Nanocrystallites Supported by Silica Nanostructures", with Z. L. Wang, Nano. Letters 1, 449-451 (2001).
- "Silica Nanotubes and Nanofiber Arrays", with R. P. Gao, Z. L. Wang, and J. D. Stout, Advanced Materials 12, 1938 (2000).
- "Side-by-Side Silicon Carbide - Silica Biaxial Nanowires - Structure, Structural Evolution and Mechanical Properties", Z. L. Wang, Z. R. Dai, Z. G. Bai, R. P. Gao, and J. L. Gole, Appl. Phys. Lett. 77, 3349 (2000).
- "Direct Synthesis of Silicon Nanowires, Silica Nanospheres, and Wire-Like Nanosphere Agglomerates", with John Stout, William Rauch, and Z. L. Wang, Applied Physics Letters 76, 2346 (2000).
- Nanocatalysis
- "Optical and Electronic Properties of Semiconducting Nanostructures for Photocatalytic Hydrogen Generation", A. Ogden, J.L. Gole, and A.G. Fedorov, Journal of Nanoelectonics and Optoelectronics, 2, 269-277 (2007).
- "Optical Properties of In-Situ-Doped and Undoped Titania Nanocatalysts and Doped Titania Sol-Gel Nanofilms", A. Ferreira da Silva, I. Pepe, J.L. Gole, S.A. Tomas, R. Palomino, C. Persson, R. Ahuja, W.M. de Azevedo, and E.F. da Sila, Jr., Applied Surface Sciences, 252, 5365 (2006).
- Reply to Comment by Gopinath on "Photoelectron Spectroscopic Investigation of Nitrogen-Doped Titania Nanoparticles" by Che, X.; Burda, C. J. Phys. Chem. B 2204, 108, 15446, (2006). C. Burda and J.L. Gole.
- "Photodegredation of Ethylene Using Visible-Light Responsive Surfaces Prepared from Titania Nanoparticle Slurries", with S. Kumar and A. G. Fedorov, Journal of Applied Catalysis B: Environmental, 57, 93-107 (2005).
- "Formation of Oxynitride as the Photocatalytic Enhancing Site in Nitrogen-doped Titania Nanocatalysts Comparison to a Commercial Nanopowder", with X. Chen, Y. Lou, A.C.S. Samia,, C. Burda, and J.L. Gole, Advanced Functional Materials, 15, 41-49 (2005).
- "Unusual Properties and Reactivity at the Nanoscale", with Clemens Burda, Z. L. Wang, and Mark White, Physics and Chemistry of Solids, 66, 546 (2005).
- "Efficient Formation of Active Silica and Doped and Metal Seeded Titania for Visible Light Tunable Photocatalysis: Application to Microreactors, Solar Cells, and Sensors", with Mark White, Andrei Fedorov, and Clemens Burda, Proceedings of TMS, Advanced Materials for Energy Conversion , Edited by Danesh Chandra, Renato Baulista, and Edwin Schaplach, Minerals, Metals, & Materials Society, pg. 69.
- "Highly Efficient Formation of Visible Light Tunable TiO2-xNx Photocatalysts and Their Transformation at the Nanoscale", J. L. Gole, J. Stout, C. Burda, Y.Lou, and X. Chen, J. Phys. Chem. 108B, 1230 (2004).
- "Enhanced Reactivity and Phase Transformation at the Nanoscale: Efficient Formation of Active Silica and Doped Metal Seeded TiO2-xNx Photocatalysts", with Clemens Burda, Andrei Fedorov, and Mark White, Reviews on Advanced Materials Science 5, 265 (2003).
- "Highly Efficient Formation of TiO2-xNx Based Photocatalysts-Potential Applications for Active Sites in Microreactors, Sensors, and Photovoltaics", with C. Burda, A. G. Fedorov and S. M. Prokes, Materials Research Society, Proceedings, Nanoparticulate Materials, 789, 422 (2003).
- "Enhanced Nitrogen Doping in TiO2 Nanoparticles", with C. Burda, Y. Lou, X. Chen, A. C. S. Samia, and John Stout, Nano Lett. 3, 1049 (2003).
- "Tunable Surface Oxidation States in Si/SiO2 Nanostructures Prepared from Silicon/Silica Mixtures and Phenol Hydroxylation Activity", J. L. Gole, B. D. Shinall, A. V. Iretskii, M. G. White, W. B. Carter, and A. S. Erickson, Chem. Phys. Chem. 4, 1016 (2003).
- "Nanocatalysis: Selective Conversion of Ethanol To Acetaldehyde Using Mono-Atomically Dispersed Copper on Silica Nanospheres", with Mark White, Journal of Catalysis 204, 249 (2001).
B. Porous Silicon-Structure, Sensors, and Microreactors and Nanotechnology
-
“Nanostructure Directed Physisorption vs. Chemisorption at Semiconductor Interfaces: The Inverse of the Hard-Soft Acid-Base (HSAB) Concept” James L. Gole and Serdar Ozdemir, Chem. Phys. Chem., in press.
-
“A Phosphine Detection Matrix Using Nanostructure Modified Porous Silicon Gas Sensors,” Serdar Ozdemir and James L. Gole, Sensors and Actuators B, in press.
-
“Porous Silicon Gas Sensors for Room Temperature Detection of Ammonia and Phosphine”, S. Ozdemir and J.L. Gole, in Chemical Sensors 8: Chemical (Gas, Ion, Bio) Sensors and Analytical Systems, ECS Transactions, Vol. 16, no. 11, pp 379-387 (2008).
- “Active Microfiltered Sensor Interfaces, Photocatalytic Reactors, and Microbatteries Using Combined Micro/nanoporous Interfaces”, James L. Gole, James Corno, Serdar Ozdemir, Sharka Prokes, and Heon-Cheol Shin, Phys. Stat. Solid, 6, 1773-1776 (2009).
- “Active Nanostructures at Interfaces for Photocatalytic Reactors and Low-power Consumption Sensors,” James L. Gole, S. Ozdemir, S.M. Prokes, and D.A. Dixon, MRS proceedings, submitted.
- "Efficient Nanostructure Modified Interfaces for Array-based Sensing Based on the Novel Application of Hard/Soft Acid/Base Interactions,” James L. Gole and S. Ozdemir, Phys. Stat. Solidi., submitted.
- “Novel Concept for the Formation of Sensitive, Selective, Rapidly Responding Conductometric Sensors,” James L. Gold and S. Ozdemir, M.R.S. Proceedings, submitted.
- “Gas Transport and Response in Porous Silicon Sensors,” Serdar Ozdemir and James L. Gole, M.R.S. Proceedings, submitted.
- "Method for the Formation of Submicrometer Copper Primer Films as the Seeding Material for Nanoelectronic Metal Contacts on Insulators," James L. Gole, Jiguang Li, and M. Schlesinger, Jour. Electrochem. Soc., submitted.
- "Active Microfiltered Sensor Interfaces, Photocatalytic Reactors, and Microbatteries Using Combined Mircro/Nanoporous Interfaces", James L. Gole, James Corno, Serdar Ozdemir, Sharka Prokes, and Heon-Cheol Shin, Phys. Stat. Solid, 6, 1773-1776 (2009).
- "The Potential of Porous Silicon Gas Sensors", Serdar Ozdemir and James L. Gole, in Current Opinions in Solid State and Materials Science, 11, 92-100 (2008).
- "Multiscale Mass Transport in Porous Silicon Gas Sensors", Peter A. Kottke, Andrei G. Fedorov, and James L. Gole, in Modern Aspects of Electrochemistry, M. Schlesinger editor, Vol. 43, pp 139-168, Springer.
- "Porous Silicon Gas Sensors for Room Temperature Detection of Ammonia and Phosphine", S. Ozdemir and J.L. Gole, in Chemical Sensors 8: Chemical (Gas, Ion, Bio) Sensors and Analytical Systems, ECS Transactions, Vol. 16, no. 11, pp 379-387 (2008).
- "A Simple Method for Generating Nano-pillars and Uniformly Separated Nano-needle Arrays on Silicon", Richard Giuly, James A. Corno, and James L. Gole, Materials Letters, 62,2704 (2008)
- "Optical Analysis of the Light Emission from Porous Silicon: A Hybrid Polyatom Surface-Coupled Fluorophor", J. L. Gole, E. Veje, E. C. Egeberg, A. Ferreira da Silva, I. Pepe and D. A. Dixon, J. Phys. Chem., 110B, 2064 (2006).
- "Pulsed System Frequency Analysis for Device Characterization and Experimental Design", J. L. Gole, S. E. Lewis and J. R. DeBoer, Sensors and Actuators B, 122, Issue 1, 20-29 (2007).
- "Porous Silicon-Sensors and Future Applications, J. L. Gole and S. E. Lewis in "Nanosilicon", Elsevier Publishers, S. Kumar, Editor, Elsevier, London, pp147-192.
- "Time-Dependent Density Functional Theory Predictions of the Vertical Excitation Energies of Silanones as Models for the Excitation Process in Porous Silicon", J. L. Gole, D. A. Dixon, J. Phys. Chem., 109B, 14830-14835 (2005).
- "Sensitive, Selective, and Tunable Nanostructure Modified Porous Silicon Gas Sensor", J.L. Gole, S. Lewis and P. Hesketh, Semiconductor Materials for Sensing, edited by Sudipa Seal, Marie-Isabelle Baraton, Clyde Parrish, and Norimitsu Murayama. Mater. Res. Soc. Symp. Proc. 828, Warrendale, PA, paper A1.7 (2005).
- "Sensitive, Selective, and Analytical Improvements to a Porous Silicon Gas Sensor", J. L. Gole, S. E. Lewis, J. R. De Boer, and P. J. Hesketh, Sensors and Actuators, B110, 54-65 (2005).
- "Nanostructure and Morphology Modified Porous Silicon Sensors", J.L. Gole, S.E. Lewis, in Quantum Sensing and Nanophotonic Devices – Nanostructures in Silicon, SPIE-Proceedings, Volume 5732, 573-583 (2005).
- "Porous Silicon Negative Electrodes for Rechargeable Lithium Batteries, Heon-Cheol S., J. Corno , J. L. Gole, and M. Liu, J. Power Sources, 139, 314-320 (2005).
- "From Nanostructures to Porous Silicon: Sensors and Photocatalytic Reactors", A. Fedorov, P. Hesketh and C. Burda, Phys. Stat. Sol. (c), 1, S2, S188-197 (2004).
- "Photoluminescence Induced Metallization for Low Resistance Contacts", in Technology Advances, Invited, MRS Bulletin 28, 263 (2003).
- "Stereolithography on Silicon for Microfluidics and Microsensor Packaging", with L. A. Tse, P. J. Hesketh, and D. W. Rosen, Microsystem Technologies 9, 319-23 (2003).
- "A Rapid, Reversible, Sensitive, and Inexpensive Gas Sensor System Based on Porous Silicon", with L. Seals, L. A. Tse, and P. J. Hesketh, in Proceedings of the Electrochemical Society 6, 13-23 (2002).
- "Stereolithography on Silicon for Microfluidics and Microsensor Packaging", in "Microfabricated Systems and MEMS-IV, Proceedings The Electrochemical Society 6, 136-143 (2002).
- "Rapid, Reversible, and Sensitive Porous Silicon Gas Sensor", with L. Seals, L. A. Tse and P. J. Hesketh, Journal of Applied Physics 91, 2519 (2002)
- "Characterization of Stereolithography Fabricated Gas Chromatographic Column", in Chemical and Biological Sensors and Analytical Methods - II, with L. A. Tse, L. Seals, D. W. Rosen, and P. J. Hesketh, Proceedings (The Electrochemical Society)18, 664-668 (2001).
- "Patterned Metalization of Porous Silicon from Electroless Solution for Direct Electrical Contact", with L. Seals and P. Lillehei, Jour. Electrochemical Society 147, 3785 (2000).
- "Contrasting Photovoltaic Response and Photoluminescence for Distinct Porous Silicon Pore Structures - A Connection Between Bulk and Surface Properties", with P. Lillihei, L. Seals, J. A. DeVincentis, and S. Narasimha, Phys. Rev. B 61, 7589 (2000).
- "Isomerization of Fluorophors on a Treated Silicon Surface", with D. A. Dixon, J. Phys. Chem. 104B, 1777 (2000).
- "Chloride Salt Enhancement and Stabilization of the Photoluminescence for a Porous Silicon Surface", with L. Seals, J. A. DeVincentis, P. Lillehei, S. M. Prokes, and D. A. Dixon, Phys. Rev. B 61, 5615 (2000).
- "Optical Pumping of Dye Complexed and Sensitized Porous Silicon to Increase Photoluminescence Emission Rates", with J. A. DeVincentis, and L. Seals, Journal of Physical Chemistry 103B, 979 (1999).
- "On the Resonantly Excited Photoluminescence from Porous Silicon and the Question of Bulk Phonon Replicates", with Sharka M. Prokes, Phys. Rev. B 58, 4761 (1998).
- "Evidence of Oxide Formation from the Single and Multiphoton Excitation of a Porous Silicon (PS) Surface or Silicon 'Nanoparticles'", J. L. Gole and D. A. Dixon, Jour. Appl. Phys. 83, 5985 (1998).
- "On the Origin of Porous Silicon Photoluminescence - Evidence for an Oxyhydride-Like Emitter", with F. Dudel, D. R. Grantier, and D. A. Dixon, Phys. Rev. B 56, 2137 (1997).
- "Stabilization of the Photoluminescence from Porous Silicon: The Competition Between Photoluminescence and Dissolution", with F. P. Dudel, J. Appl. Phys. 82, 402 (1997).
C. Lithium Microbatteries
- "Microstructured Nanopore-walled Porous Silicon as an Anode Material for Rechargeable Lithium Batteries", Dae-Geun Kang, James A. Corno, James L. Gole, and Heon-Cheol Shin, Journal of Electrochemical Society, 155, A276-A281 (2008).
- "Porous Silicon Negative Electrodes for Rechargeable Lithium Batteries", S. Heon-Cheo, J. Corno, J.L. Gole and M. Liu, J. Power Sources, 139, 314-320 (2005).
- "Porous Silicon-Based Electrodes for Lithium Batteries", with H.-C. Shin, Z. Shi, L. T. Seals, and M. Liu, J.L. Gole, Solid State Ionic Devices III, Edited by E. Wachman et al., Proceedings of the Electrochemical Society, Pennington, NJ, Vol. 2002-26, pp. 518-525 (2003).
- "Generation of Highly Porous LiMg and LiZn Alloys from Kinetically Controlled Lithiation", with Zhong Shi and Meilin Liu, Philosophical Magazine B81, 119-131 (2001).
- "Electrochemical Properties of Li-Mg Alloy Electrodes for Lithium Batteries", with Zhong Shi, Devang Naik, and Meilin Liu, Journal of Power Sources 92, 70 (2001).
- "Electrochemical Properties of Zn-Li Alloy Electrodes Prepared by Kinetically Controlled Vapor Deposition for Lithium Batteries", with Zhong Shi and Meilin Liu, Electrochemical and Solid State Letters 3, 312 (2000).
- "Kinetically Controlled Lithiation: A Variant of Physical Vapor Deposition with Application to Lightweight Alloys and Lithium Batteries", with F. Dudel, T. A. Sanders, J. King, and R. F. Browner, Philosophical Magazine B75, 733-755 (1997).
D. Select Chemical Physics
- "Structure and Heats of Formation of Simple Alkali Metal Compounds: Hydrides, Chlorides, Fluorides, Hydroxides, and Oxides for Li, Na, and K." M. Vasiliu, S. Li, K.A. Peterson, J.L. Gole, and D.A. Dixon, J. Phys. Chem. A, 114, 4272 (2010).
- "Molecular Electronic Structure, Electronic Spectra, Ionization Potential, and Ground State Spin Orbit Splitting of Si2N", with S.J. Paukstis, D.A. Dixon, and K.A. Peterson, J. Chem. Phys., submitted.
- "Time-Dependent Density Functional Theory Predictions of the Vertical Excitation Energies of Silanones as Models for the Excitation Process in Porous Silicon", J. L. Gole, D. A. Dixon, J. Phys. Chem., B109, 14830 (2005).
- "The Ionization Potential of Si2N and Si2O", with S. J. Paukstis, D. A. Dixon, and K. A. Peterson, J. Phys. Chem., Setzer Festschift, submitted to J. Phys. Chem. A106, 8435 (2002).
- "Measurement of Low-Level Gain in a Visible Chemical Laser Amplifier", with J. M. Stephens, J. Stout, J. Abraham, M. Neumann, and L. A. Sentman, Opt. Eng. 40 (12) 2788-2801 (December 2001).
- "Ultrafast Energy Transfer and Population Buildup Among Strongly Coupled and Curve Crossing Regions of the Boron Oxide A2P and X2S+ States", with Bengt Ohllson and Gary Green, Chemical Physics 273, 59-72 (2001).
- "Excited State Mediated Collision Induced Raman Pumping", with D. R. Grantier, Molecular Physics 98, 1441 (2000).