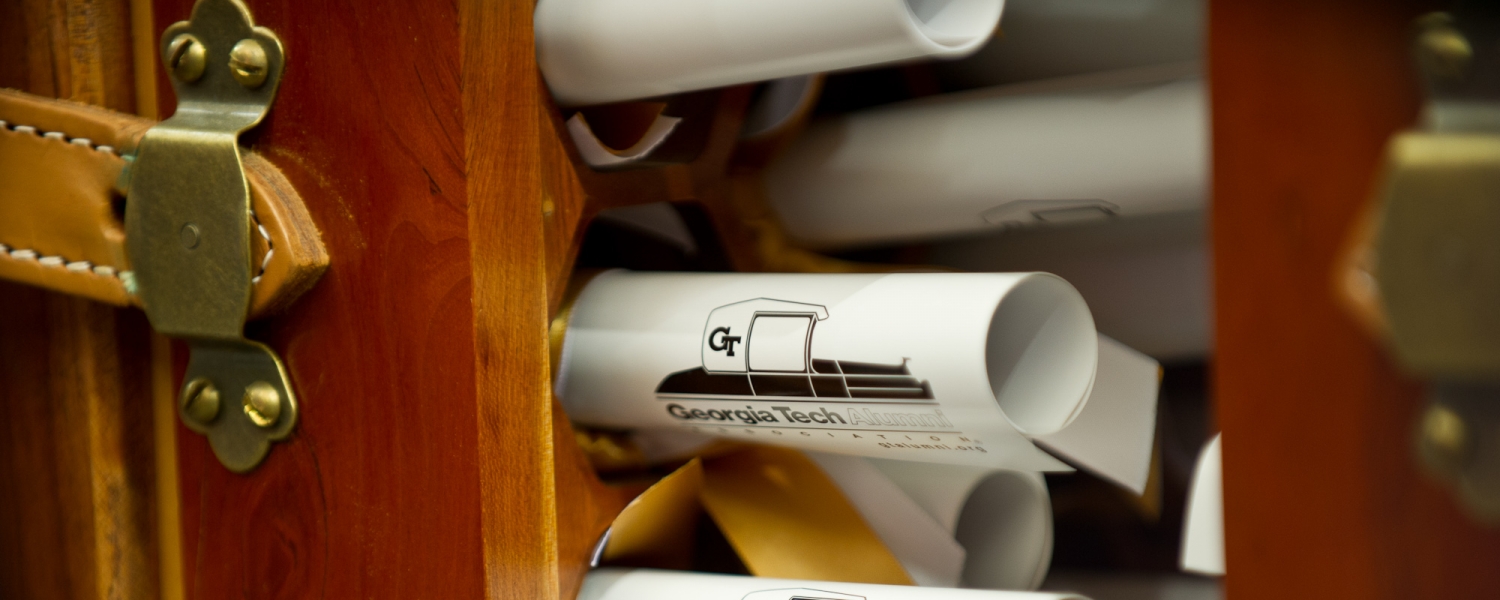
Motivated by issues related to treating certain neurological diseases such as Parkinson’s disease by a method called electrical deep brain stimulation, we consider applying optimal control methods to both mathematical models of neurons and in vitro neurons. Patients suffering from Parkinson’s disease experience involuntary tremors that typically affect the distal portion of their upper limbs. It has been hypothesized that these tremors are associated with simultaneous spiking of a cluster of neurons in the thalamus and basal ganglia regions of the brain. In a healthy situation, the periodic firing of neurons is not synchronized, but they can engage in a pathological synchrony and all fire at the same time which results in release of strong action potentials that trigger the downstream muscles with periodic shocks, manifested as tremors.
In this talk, we investigate the control of different neuronal systems using methods of optimal control. The neuronal systems considered range from simple one-dimensional phase models to multi-dimensional conductance-based models, both on a single neuron level and on a population level. The optimal control methods considered produce event-based, continuous-time, typically bounded input stimuli that can optimally achieve the desired control objective. The optimality criterion considered is minimum energy. The control objectives of interest are the interspike interval for single neurons and desynchrony for populations of neurons. The applicability of the interspike interval controller is shown in practice by testing it on single in vitro pyramidal neurons in the CA1 region of rat hippocampus.
Event Details
Date/Time:
I will discuss three examples describing the utility of understanding and/or exploiting both epigenetic and genetic variability in populations of yeast cells. First, I describe an unappreciated and dominant role for cell-cycle phase on transcriptional variability and dynamics. We show that for a model “noisy” gene in S. cerevisiae, the common view that large variability observed in mRNA numbers is due to a transcriptional bursting, where a promoter undergoes random and intermittent periods of active transcription, is incomplete and possibly incorrect. Rather, variable mRNA distributions are largely driven by differences in transcriptional activity between G1 and S/G2/M phases of the cell cycle. The cell-cycle phase is also paramount when probing variability in the kinetics of gene activation, with early S/G2 appearing to be far more permissive for activation. This global cell-cycle dependence may be essential to consider when using stochastic models to predict the behavior of both natural and synthetically engineered gene networks, especially in conditions when growth rate changes.
Second, I describe how variable numbers of tandemly repeated “decoy” transcription factor (TF) binding sites that bind a cognate transcriptional activator can reduce expression at the activator’s target genes, qualitatively converting the dose-response from a linear to steeper sigmoidal-like threshold response. The results imply that 1) transcription factor (TF) / promoter binding may be weaker than expected in the context of gene expression and 2) there may be a previously unappreciated negative cooperativity in TF binding to clustered sites. Therefore, even small quantitative changes in the highly variable length of repetitive DNA containing TF binding sites found in eukaryotic genomes can have qualitative effects on gene expression, and perhaps ultimately phenotype.
Third, I describe a technique we have developed for the repeated and targeted in vivo mutagenesis of multiple genes in S. cerevisiae. We show selective damage of DNA and subsequent repair by error-prone homologous recombination pathways can lead to selective >800-fold increase in mutation rate in a user-defined 20 kb target region. We discuss applications of this method for the directed evolution of multigenic phenotypes. Deployment is simple and our constructs and protocols are available to interested researchers.
Event Details
Date/Time:
Multicellular behavior in bacterial biofilms is intimately tied to the production of an extracellular polysaccharide (EPS) matrix that encases the cells and provides physical integrity to the colony as a whole. As a colony grows from a few cells into a biofilm, a sudden increase in EPS production generates osmotic stresses that cause the biofilm to expand. Moreover, EPS production is triggered by a nutrient depletion gradient that develops in the biofilm due to diffusive mass transport limitations. These polymer physics based biofilm behaviors suggest that EPS production may have evolved in biofilms to beat the diffusion limit of nutrient transport into the colony, though no direct observation of nutrient transport has been observed previously. In this talk I will discuss measurements of nutrient transport into b. subtilis biofilms and show that when EPS production is up-regulated, the polymer sucks fluid into the colony with a characteristic time dependence like that of pressure driven flow.
In contrast to bacteria in biofilms, eukaryotic cell behavior in tissues is intimately tied to forces generated by molecular motor-driven contractions. Contraction generated tensions are balanced by deformations in the cell's microenvironment, by internal cytoskeletal structures, and by the incompressible cytosolic fluid contained within the cell membrane. However, contraction generated pressures cannot be supported by the cytosol if the cell membrane is adequately permeable. Small, non-selective pores called gap junctions connect cells in a layer, allowing small molecules to pass between cells. In the second half of this talk I will discuss measurements of contraction driven fluid movement across gap junctions connecting neighboring cells. We observe contracting cells pushing fluid into their neighbors. To study the mechanics of intercellular fluid flow, we apply biologically relevant pressures to large regions of cells in a monolayer with a micro-indentation system. We directly measure indentation force and volume as a function of time to determine fluid flow rates and associated stresses between cells. We find that gap-junction permeability does not limit fluid transport between cells, and that fluid flow is controlled by a balance of cytoskeletal tension throughout the cell monolayer.
Event Details
Date/Time:
The motor protein kinesin uses adenosine triphosphate (ATP) as a fuel and walks along the microtubule filaments in the cell. They are vital for many
cellular processes including intracellular transport and cell division. Although recent progress in experiments yielded much information regarding
their motility, a structure-based, physical mechanism by which the motor amplifies the ATP-driven small conformational change in the motor head into
a large, 8-nm stepping motion remains largely a mystery. I will discuss molecular dynamics simulations elucidating its force-generation mechanism
and tests by single-molecule optical trap measurements. We find that the motion consists of autonomous force-generation and guided diffusion, where
the partitioning of the two depends on functional needs of different kinesin families.
Event Details
Date/Time:
I will describe several models for running insects, from an energy-conserving biped, through a muscle-actuated hexapod driven by a neural central pattern generator, to a reduced phase-oscillator model that captures the dynamics of unperturbed gaits and of impulsive perturbations. I will argue that both simple models and large simulations are necessary to understand biological systems. The models show that piecewise-holonomic constraints due to intermittent foot contacts confer asymptotic stability on the feedforward system, while leg force sensors modulate motor outputs to mitigate large perturbations. Phase response curves and coupling functions help explain reflexive feedback mechanisms. The talk will draw on joint work with Einat Fuchs, Robert Full, Raffaele Ghigliazza, Raghu Kukillaya, Josh Proctor, John Schmitt, and Justin Seipel. Research supported by NSF and the J. Insley Blair Pyne Fund of Princeton University.
Bio:
Philip Holmes was born in England in 1945 and educated at the Universities of Oxford and Southampton. He taught at Cornell from 1977 to 1994, when he moved to Princeton, where he is a Professor of Mechanical and Aerospace Engineering and of Applied and Computational Mathematics and a member of Princeton's Neuroscience Institute. Much of his research has been in dynamical systems and their applications in engineering and the physical sciences, but in the past 15 years he has increasingly turned to biology. He currently works on animal locomotion and the neuro-dynamics of decision making. He has also published four collections of poems (Anvil Press, London).
Event Details
Date/Time:
Given the complexity of the dynamics of a plasma, reductions are needed. The Hamiltonian framework offers various ways to carry out such reductions. I will introduce some of these methods by considering in particular the example of gyrokinetics, which is a kinetic model for plasmas in a strong magnetic field.
Event Details
Date/Time:
In this seminar, we will discuss how interactions between discrete electromagnetic (EM) emitters lead to a cooperative response of a metamaterial or cold atomic gas to an incident field. We will examine a number of ways the cooperative response can be engineered and exploited. The interactions responsible arise from repeated scattering of the field between its discrete constituent elements. Our focus will be on metamaterials, which are composed of subwavelength circuit elements - or meta-atoms - whose electric and magnetic multipole interactions are governed by their design. We also apply these ideas to atoms in an optical lattice. Understanding cooperative interactions between cold atoms may be useful, for example, in the further enhancement of quantum memories.
Event Details
Date/Time:
The defining feature of a black hole is its event horizon through which nothing can escape outward, not even light. And yet black holes are responsible for the brightest sources that we observe in the universe, from gamma-ray bursts to quasars. Their enormous luminosity arises from the release of gravitational binding energy outside the horizon as material falls inward. These black hole accretion flows exhibit extraordinary behavior, including outbursts, state transitions, and quasi-periodic variability, most of which continue to defy understanding but which must be related to the magnetohydrodynamic turbulence and radioactive thermodynamics that govern the physics of these flows. I will review recent theoretical progress in understanding this physics, much of which has been achieved through numerical simulations of the flows.
Event Details
Date/Time:
Despite their everyday familiarity, thin sheets (paper, plastic, fabric, etc.) display remarkable and complex behaviors that still challenge theoretical description. The intricate coupling between the geometry of surfaces and the elasticity of a thin sheet necessarily leads to the formation of singularities, nonlinear elasticity, and geometric frustration. Nevertheless, multicellular organisms - like you - develop their three dimensional structures in part by exploiting these elastic phenomena. These considerations have led to new theoretical and experimental tools to shape elastic sheets into prescribed 3D shapes using the principles of non-Euclidean geometry. I will describe our attempts to design sheets that fold controllably into 3D structures and some related problems in the mechanics of origami, where 3D structure is developed by folding a piece of paper. These techniques open up new avenues in "experimental mathematics", allowing us to explore geometry experimentally.
Event Details
Date/Time:
What could possibly be new in the Ising model, arguably the most-studied model of statistical physics? Plenty! Consider the Ising model initially at
infinite temperature that is suddenly cooled to zero temperature and evolves by single spin-flip dynamics. What happens? In one dimension, the ground state is always reached and the evolution can be solved exactly. In two dimensions, the ground state is reached only about 2/3 of the time, and the long-time evolution is characterized by two distinct time scales, the longer of which arises from topological defects. In three dimensions, the ground state is never reached and the evolution is quite rich: (i) domains are topologically complex, with average genus growing algebraically with system size; (ii) the long-time state always contains "blinker" spins that can flip ad infinitum with no energy cost; (iii) the relaxation time grows
exponentially with system size.
Event Details
Date/Time:
Pages
