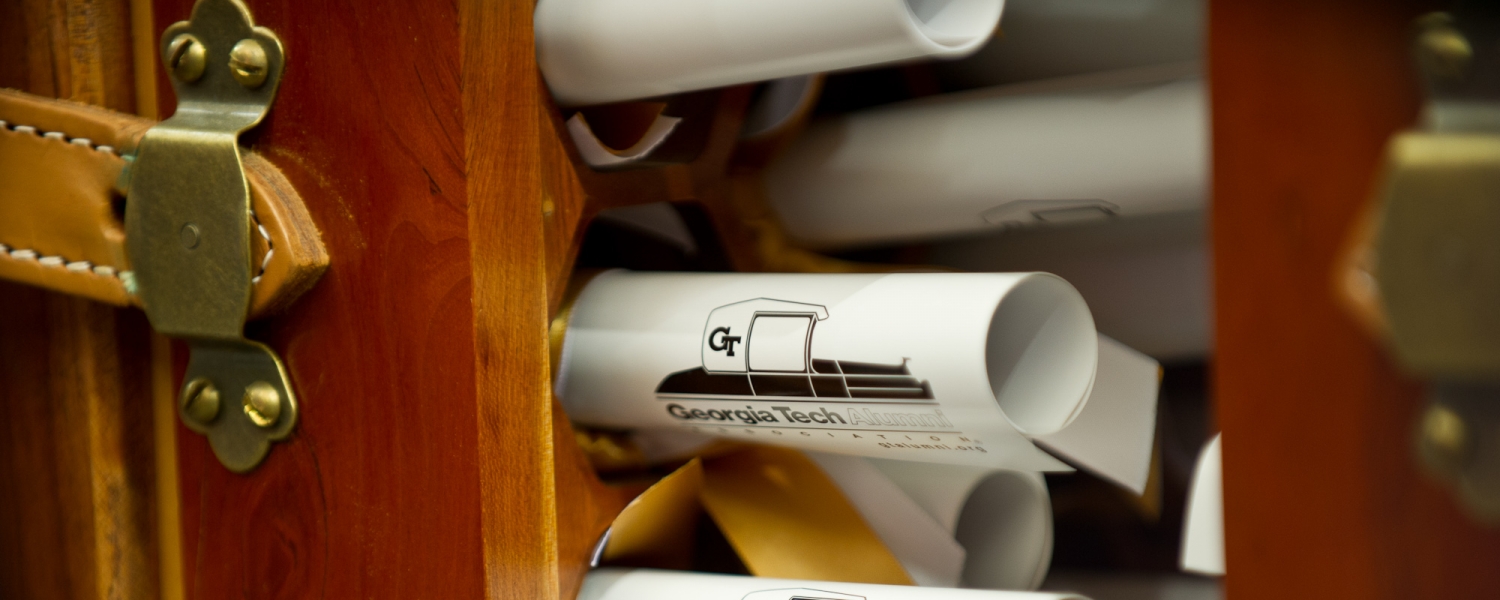
Abstract:
Emergence is a ubiquitous feature of quantum condensed matter systems: the collective low-energy behavior of an interacting quantum many-body system oftentimes exhibits behavior profoundly different from that of the constituent degrees of freedom. In this talk, I will present a survey of recent results on one- and two-dimensional quantum systems which dramatically demonstrate this concept. In the first part of the talk, I will focus on a set of problems in which we are able to *uncover* — through both computational and analytical lines of attack — novel and striking emergent behavior in (1) the kagome Heisenberg antiferromagnet and (2) the half-filled Landau level. I will discuss the emergence and entanglement structure of fermionic spinons and composite fermions, respectively, in these two paradigmatic quantum condensed matter systems.
In the last part of the talk, I will switch gears and discuss how we can *exploit* emergent quantum phenomena for technological gain. In particular, I will discuss how Majorana fermions can emerge as zero-energy features of certain superconducting wires and how these Majorana zero modes can in principle be used to build superior quantum computing hardware: In this so-called topological approach to quantum computation, Majorana-based qubits remarkably allow perfect insensitivity to local noise as well as implementation of perfect quantum gates. Focusing on the former property, I will discuss our recent proposals for verifying topological (“perfect”) protection of quantum information in present-day devices being pursued vigorously by experimental groups at Microsoft and elsewhere. I will conclude by discussing several future directions of research in these areas.
Event Details
Date/Time:
Abstract:
Quantum many-body systems are difficult to study because the space of possible many-body states is huge: its dimension grows exponentially in the system size. However, in recent years progress in our understanding of quantum entanglement has revealed that only a small region of this huge state space is actually relevant to the study of quantum many-body systems.
Tensor networks are a powerful formalism developed to efficiently describe the states in this small, physically relevant region of the many-body state space. In this Colloquium I will (i) review our current understanding of many-body entanglement, (ii) introduce tensor networks as an efficient description of many-body states, and (iii) give an overview of the exciting developments within the tensor network program.
Event Details
Date/Time:
Abstract: The exact solution to interacting quantum problems is, in general, an exponentially hard task due to the exponential growth of the Hilbert space with the system size. As a result, despite extensive research during the past several decades we still do not have a good understanding of strongly correlated systems even for the simplest ones such as the Hubbard model. We can only obtain exact results for special and very limited classes of models. For example, quantum Monte Carlo (QMC) method which evaluates path integrals stochastically is an exact and unbiased numerical method provided the notorious sign-problem is absent.
In this talk, I will introduce our recently developed algorithm that can find the reduced density matrix associated with a finite subsystem of an infinite system. This paradigm was inspired by ideas from Hawking-Unruh radiation in black hole physics. As an example, I will demonstrate that this method can obtain the exact ground-state energy and scaling dimensions of the 1D Heisenberg model in the thermodynamic limit by solving a few sites problem. I will then show that within this new approach, the sign problem is practically circumvented and QMC can be applied to generic local models even at extremely low temperatures. I will finally show that this paradigm can also enhance the accuracy of matrix/tensor product states significantly by allowing gigantic bond dimensions.
Event Details
Date/Time:
Abstract:
The study of liquid crystals occupies a central place in materials science, serving as a context for encountering and using a variety of exotic structural themes of molecular organization, particularly of soft matter. A key feature of molecular ordering in liquid crystals is fluid hierarchical self-assembly, in which molecular structure provides precise control of fluid self-organization over a wide range of length scales.
A notable outcome of such behavior has been the development of liquid crystal displays, a key enabling technology of the portable computing revolution of the twentieth century. I will present some self-assembly themes of current interest in liquid crystal, colloids, clays, and films, and argue that liquid crystal hierarchical self-assembly may have been a key feature of early life.
Event Details
Date/Time:
Abstract:
Animals are capable of performing an amazing set of actions. In many species, particularly invertebrates (although scarily also humans), a large percentage of the behavioral repertoire is comprised of stereotyped behaviors—actions that are seen again and again across time and individuals. I will discuss our recent work trying to uncover how these behaviors are actuated and controlled in the wiggling nematode worm C. elegans and the dancing fruit fly D. melanogaster.
Event Details
Date/Time:
Abstract:
The simple process of crumpling a sheet of paper with our hands results in a complex network of interconnected permanent creases of many sizes and orientations. Sheet preferentially bends along these creases, introducing history dependence to the process of crumpling.
I will present an experimental study of the dynamics of crumpling. Specifically, I will first discuss how a crease network evolves when a thin elastoplastic sheet is repeatedly crumpled, opened up and then re-crumpled. Is there a maximally crumpled state after which the flat sheet can be deformed without further plastic damage? If time permits I will also I'll discuss the crumpling of soda cans, focusing on our attempts to characterize their stability in the case where on single defect dominates buckling.
Event Details
Date/Time:
Exploring the physics of spontaneous emission with an artificial atomic system
Abstract:
The question how irreversibility can emerge in quantum mechanics is central to the study of open quantum systems. A simple example is the exponential decay of an excited two-level atom as described by the Wigner-Weisskopf model of quantum optics, in which spontaneous photon emission is sometimes viewed as being driven by fluctuations of the surrounding vacuum. However, the Markov approximation underlying this model can be violated under certain conditions, and recent experiments on optical decay in photonic bandgap materials have indeed started to find deviations from its predictions.
We have recently realized an "artificial atom" in which the excited-state energy and the vacuum coupling can be controlled at will, thus allowing for a systematic exploration of spontaneous emission beyond the Markov approximation. Naively, a two-level atom may be viewed as a photon trap that is empty or full; we instead realize a microscopic trap for a single atom that can decay, under coherent external driving, by emitting the atom into the surrounding vacuum. The experiments are performed using an optical lattice geometry that provides arrays of such artificial atoms coupled to a one-dimensional matter waveguide. In my talk I will present experimental results on Markovian and strongly non-Markovian dynamics in this system, including exponential and partly reversible oscillatory decay, atom reabsorption, as well as a bound state for emission below the edge of the mode continuum that features an evanescent matter wave and which is the direct analog of the long-predicted atom-photon bound state in photonic bandgap materials. I will conclude with an outlook on future applications of our system in open-system many-body quantum physics.
Event Details
Date/Time:
Abstract:
Although scanning tunneling microscopy (STM) first gave us a real-space atomic-scale image of the silicon surface over 30 years ago, the ability to make electrically useful devices atom-by-atom has just recently advanced to the point where it can start being used as a tool to address basic science questions and engineering challenges. We have recently directed our ability to make atomically precise donor structures in silicon to fabricating tunable arrays that hold promise for serving as small-scale analog quantum simulations of quantum material systems. This would establish an engineered approach to quantum materials, complementary to trapped atoms and ions. This talk will focus both on the promise of realizing quantum materials in tunable donor arrays, and on our technical progress towards that goal.
Event Details
Date/Time:
Abstract
Liquid crystal elastomers combine the orientational order of liquid crystals with the elasticity of polymers. Remarkably, these materials flex and deform under stimuli such as a change of temperature, and undergo autonomous folding, or "auto-origami," into complex shapes. The material's liquid crystal director field defines the local axis of extension/contraction, and can be patterned, or "blueprinted," to induce a programmed shape transformation. Incorporation of photoactive azobenzene makes these materials move in response to illumination.
We model the dynamics of these shape transformations using finite element elastodynamics, examining director fields incorporating twist, splay, and high-order topological defects. We also model the generation of light-driven mechanical wave motion in a photoactive liquid crystal polymer film [1], in collaboration with the Broer experimental group at TU Eindhoven.
Our simulations demonstrate the mechanism that produces continuous, directional, macroscopic mechanical waves under constant light illumination, with a feedback loop driven by self-shadowing. Potential applications include autonomous light-driven locomotion and self-cleaning surfaces.
Work supported by NSF-DMR 1409658, NSF-CMMI 1436565, and NSF-CMMI 1663041. [1] Anne Helene Gelebart, Dirk Jan Mulder, Michael Varga, Andrew Konya, Ghislaine Vantomme, E. W. Meijer, Robin L. B. Selinger, and Dirk J. Broer, "Making waves in a photoactive polymer film," Nature v. 546, p. 632, 29 JUNE 2017.
Event Details
Date/Time:
Abstract
Soft materials with orientational order can undergo dramatic shape transformations driven by change of temperature or other stimuli. Nematic elastomers, a form of liquid crystal polymer, have been patterned with topological defects and deform from a flat film into twisted, bent, folded, and curved shapes on heating or cooling. Lipid vesicles, during a phase transition from an untilted to a tilted phase, deform from smooth spheres to crumpled, disordered shapes. In both of these materials, topological defects play a key role: they drive shape change by inducing curvature.
Conversely, a liquid crystal enclosed in a confined geometry may have topological defects even in its lowest energy state, induced by imposed curvature. We categorize these various material systems into three classes: 1. Microstructure fixed and shape evolves; 2. Shape fixed and microstructure evolves; and 3. Both shape and microstructure evolve with competing kinetics. We explore mechanisms by which each of these processes can give rise to a deterministic shape transformation or else get trapped in long-lived metastable states.
To explore these pattern-formation processes, we use simulation techniques including coarse-grained particle-based models of lipid membranes, nonlinear finite element simulation of elastic solids, continuum models of liquid crystal textures, and statistical physics models of defects in curved geometries, comparing to relevant experiments.
Work supported by NSF-DMR-1409658, and NSF-CMMI 1436565, and NSF-CMMI 1663041.
Event Details
Date/Time:
Pages
