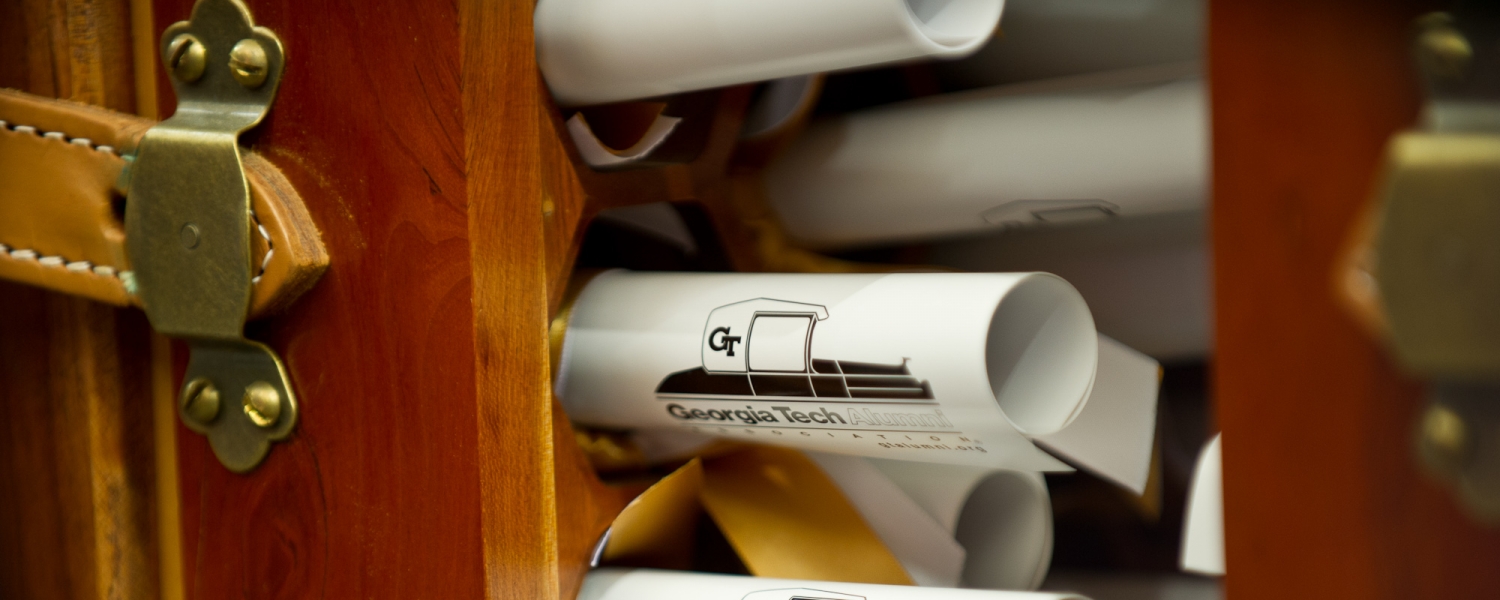
TBD
Event Details
Date/Time:
mso-fareast-font-family:"Times New Roman"">Since the 2001 Nobel Physics Prize was awarded for the creation of Bose-Einstein condensates, dilute atomic gases at ultralow temperatures have been a driving force behind the quantum simulation of manybody physics. However, studying highly correlated quantum states with small energy gaps can still pose severe challenges to contemporary experiments with even the coldest atomic samples. The power of cold-atom experiments will be greatly enhanced by precision measurements, allowing, for example, physics that is normally probed at nK temperatures to be studied at μK temperatures. This is precisely what we have achieved. Thanks to the development of ultrastable lasers with 1×10-16 instability, the JILA strontium (Sr) optical clock now realizes a powerful laboratory to study a many-body spin system with strongly interacting, open, and driven dynamics [1]. For the first time, s- and p-wave inter-atomic interactions in the clock are characterized to high precision, which enables a spectroscopic observation of SU(N
mso-fareast-font-family:"Times New Roman";mso-hansi-font-family:"Times New Roman";
mso-char-type:symbol;mso-symbol-font-family:Mathematica1">£
mso-fareast-font-family:"Times New Roman""> 10) symmetry in 87Sr gases at μK temperatures [2]. This study lays the groundwork for pushing the frontier of emergent many-body quantum physics beyond experimental limitations, as well as realizing exotic quantum states that have no counterparts in nature.
mso-fareast-font-family:"Times New Roman"">To go beyond current experimental capabilities, one will need to combine the power of precision measurements with state-of-the-art cold-atom techniques to cool, probe, and manipulate atomic quantum gases. High-spatial-resolution imaging is one such technique, which has been utilized in the observation of quantum criticality with two-dimensional Bose gases in optical lattices [3]. In this experiment, high-resolution imaging allows one not only to access the equation of state and dynamics of a quantum gas, but also to engineer arbitrary trapping potentials for studying novel quantum transport phenomena. Based on my experiences with both ultracold atoms and precision measurements, I will discuss my future research plans and explain how ultracold strontium atoms with optical flux lattices will provide a unique opportunity to explore some of the most interesting strongly correlated quantum systems.
mso-fareast-font-family:"Times New Roman"">
mso-fareast-font-family:"Times New Roman"">[1] A quantum many-body spin system in an optical lattice clock.
mso-fareast-font-family:"Times New Roman"">M. J. Martin, M. Bishof, M. D. Swallows, X. Zhang, C. Benko, J. von-Stecher, A. V. Gorshkov, A. M. Rey, and J. Ye, Science 341, 632 (2013).
[2] Spectroscopic observation of SU(N)-symmetric interactions in Sr orbital magnetism.
X. Zhang, M. Bishof, S. L. Bromley, C.V. Kraus, M. Safronova, P. Zoller, A. M. Rey, and J. Ye, mso-fareast-language:ZH-CN">Science 345, 1467 (2014).
[3] ZH-CN">Observation of quantum criticality with ultracold atoms in optical lattices mso-fareast-font-family:"Times New Roman"">.
X. Zhang, C.-L. Hung, S.-K. Tung, and C. Chin, Science 335, 1070 (2012).
Event Details
Date/Time:
Our method of nanoscale magnetic sensing and imaging makes use of nitrogen-vacancy (NV) color centers a few nanometers below the surface of a diamond crystal. Using individual NV centers, we perform NMR experiments on single protein molecules, labeled with ^{13}C and ^2H isotopes. In order to achieve single nuclear-spin sensitivity, we use isolated electronic-spin quantum bits (qubits), that are present of the diamond surface, as magnetic resonance "reporters". Their quantum state is coherently manipulated and measured optically via a proximal NV center. This system is used for sensing, coherent coupling, and imaging of individual proton spins on the diamond surface with angstrom resolution. Our approach may enable direct structural imaging of complex molecules that cannot be accessed from bulk studies, and realizes a new platform for probing novel materials, and manipulation of complex spin systems on surfaces.
Event Details
Date/Time:
Event Details
Date/Time:
A trial wave function \f (1,2,...,N) of an N electron system can always be written as the product of an antisymmetric Fermion factor F {Zij }= Tii<jZij , and a symmetric correlation factor G {Zij }. F results from Pauli principle, and G is caused by Coulomb interactions. One can represent G diagrammatically ( I J by distributing N points on the circumference of a circle, and drawing appropriate lines representing correlation factors (cfs) Zij between pairs. Here, of course, Zij = Zi Zj, and Zi is the complex coordinate of the i111 electron. Laughlin correlation for the v=l/3 filled
For any one partition(A,B)the contribution to G is given by GAB = Tii
For example, the highest power of any Zi cannot exceed 2e+ 1-N. In addition, the value of the total angular momentum L of the lowest correlated state must satisfy the equation L=(N / 2) (2e+ 1-N)-Ka, where Ka is the degree of the homogeneous polynomial generated by G. Knowing the values of L for IQL states (and for states containing a few quasielectrons or a few quasiholes) from Jain's mean field CF picture allows one to determine Ka. The dependence of the pair pseudopotential V(L2) on pair angular momentum L2 , suggests a small number of correlation diagrams for a given value of the total angular momentum L. Correlation diagrams and correlation functions for
[1] J.J. Quinn, Waves in random and complex media (2014) 898867
[2] S.B. Mulay, J.J . Quinn, and M.A. Shattuck, submitted to J. Math. Phys. (2014)
Event Details
Date/Time:
Conventional methods of quantum simulation rely on kinectic energy determined by free particle dispersions or simple sinusoidal optical lattices. Solid state systems, by contrast, exhibit a plethora of band structures which differ quantitatively, qualitatively, and even topologically. To what extent does this variety explain the many electronic phenomena observed in these materials? Here we address this question by subjecting an otherwise simple Bose superfluid to a customized band structure engineered by dynamically phase modulating (shaking) an optical lattice. The engineered dispersion contains two minima which we associate to a pseudospin degree of freedom. Surprisingly, in such a system the Bose superfluid exhibits many new behaviors. The psuedospin develops a ferromagnetic order, which can lead to polarization of the entire sample or to sub-division into polarized domains. The excitations of the system also exhibit the roton-maxon structure associated with strong interactions in superfluid helium.
Event Details
Date/Time:
Cold atoms and ions provide an interesting playground for a variety of measurements of fundamental physics. Using RF traps, experiments become possible with both large ensembles of ions, e.g. in cold chemistry, and few/single ions, such as in quantum computations/simulations or optical clocks, where ultimate quantum control is required. In the first part of the talk, recent results from our work on cold chemistry and cold molecular ions using a hybrid atom--ion experiment will be presented. We have developed an integrated time-of-flight mass spectrometer, which allows for the analysis of the complete ion ensemble with isotopic resolution. Using this new setup, we have significantly enhanced previous studies of cold reactions in our system. Potential routes towards ultra-cold reactions at the quantum level will be presented. Current work aims at demonstrating rotational cooling of molecular ions and photo-associating molecular ions.
The second part of the talk reports on our results of the search for the low-energy isomeric transition in thorium-229. This transition in the vacuum-ultraviolet regime (around 7.8 eV) has a lifetime of tens of minutes to several hours and is better isolated from the environment than electronic transitions. This makes it a very promising candidate for future precision experiments, such as a nuclear clock or tests of variation of fundamental constants, which could outperform implementations based on electronic transitions. Our approach of a direct search for the nuclear transition uses thorium-doped crystals and, in a first experiment, synchrotron radiation (ALS, LBNL) to drive this transition. We were able to exclude a large region of possible transition frequencies and lifetimes. Currently, we continue our efforts with enhanced sensitivity using a pulsed VUV laser system.
Event Details
Date/Time:
Superfluidity, or flow without resistance, is a macroscopic quantum effect that is present in a multitude of systems, including liquid helium, superconductors, and ultra-cold atomic gases. Here, I will present our work studying superfluid flow in a Bose-Einstein condensate (BEC) of sodium atoms. By manipulating optical potentials, we are able to form BECs into any shape, including rings and targets. Ring condensates are unique in that they can support quantized, persistent currents. We drive transitions between persistent current states using a rotating perturbation, or weak link. This ring and rotating potential form a circuit, which is analogous to an rf superconducting quantum interference device (SQIUD). Our circuit shows the essential features of an rf-SQUID, including tunable transitions between quantized persistent current states and hysteresis. Such features make an rf-SQUID a sensitive magnetometer; by analogy, our device could act as a rotation sensor. In addition to these experiments, we have also realized other geometries such as a dumbbell and a dc-SQUID, that allow us to study critical velocities and resistive flow in superfluids. These, and similar experiments with tunable geometries, shed new light onto the details of quantum transport and superfluidity, and may pave the way for new ‘atomtronic’ devices.
Event Details
Date/Time:
The integer quantum Hall effect (IQHE) is often described in terms of skipping orbits: 2-D electrons begin nascent cyclotron orbits, only to be interrupted by the material’s boundary, and instead reflect from the edge, beginning a partial orbit anew. These classical skipping trajectories follow the systems boundary, and in the quantum limit connect to the quantum Hall system’s conducting edge channels. Even though decades of measurements have confirmed this overall picture, the intrinsic difficulty of imaging electrons has precluded all attempts to image these orbits.
We have directly realized a cold-atom lattice in the extreme quantum limit, where each lattice plaquette contains about 4/3 of the quantum of flux: unthinkable in crystalline materials (where it would take 104 T fields), but achievable using engineered tunneling phases in an optical lattice. To achieve such fields, we use a hybrid lattice geometry, where one dimension of the lattice is a normal optical lattice and the other dimension is the internal hyperfine states of the atoms; this gives us perfect single-site imaging resolution along one dimension. We then dynamically prepared our ultracold bosons in several different initial states, which allowed us to observe edge and bulk properties in two ways: (1) we directly imaged the edge states (associated with quantized conductance in IQHE systems) and the localized bulk states (associated with the insulating bulk of IQHE systems); (2) we then created excitations, those on the edge were analogues to edge magnetoplasmons in quantum Hall systems and directly follow their chirally oriented skipping orbits.
Event Details
Date/Time:
The emergence of attosecond spectroscopy has offered researchers a new experimental tool, which provides unprecedented temporal resolution for the direct measurement and control of electronic processes. Pioneering studies have obtained unique physical insights by taking snapshots of atomic and molecular phenomena on the natural timescale of electrons. Ongoing efforts aim to expand the scope of attosecond XUV research for exploration of complex dynamics in molecules and materials. My lab has conducted frontline investigations to probe the electronic complexity stemming from coupled nuclear motion, electronic correlations, external fields, or a combination thereof. I will present our latest results on the coherent evolution of electron hole near a conical intersection of a polyatomic molecule. Such conical intersections induce nuclear-motion-mediated electron dynamics and serve as nature’s energy funnels in many biochemical processes (e.g. DNA repair, light harvesting etc.). In a similar context, we have investigated electron-phonon coupling and electronic correlations in carbon nanomaterials. I will show that apart from the sensitive measurements of electronic couplings, we can also monitor the evolution of quantum coherence in charge transfer processes. Finally, I will discuss our ongoing work on the generation of ‘isolated’ attosecond pulses and their application in study of correlation-driven charge migration in molecules and magnetic materials. Such interdisciplinary efforts will open the attosecond field for a broad set of applications in physics, chemistry and material sciences.
Event Details
Date/Time:
Pages
