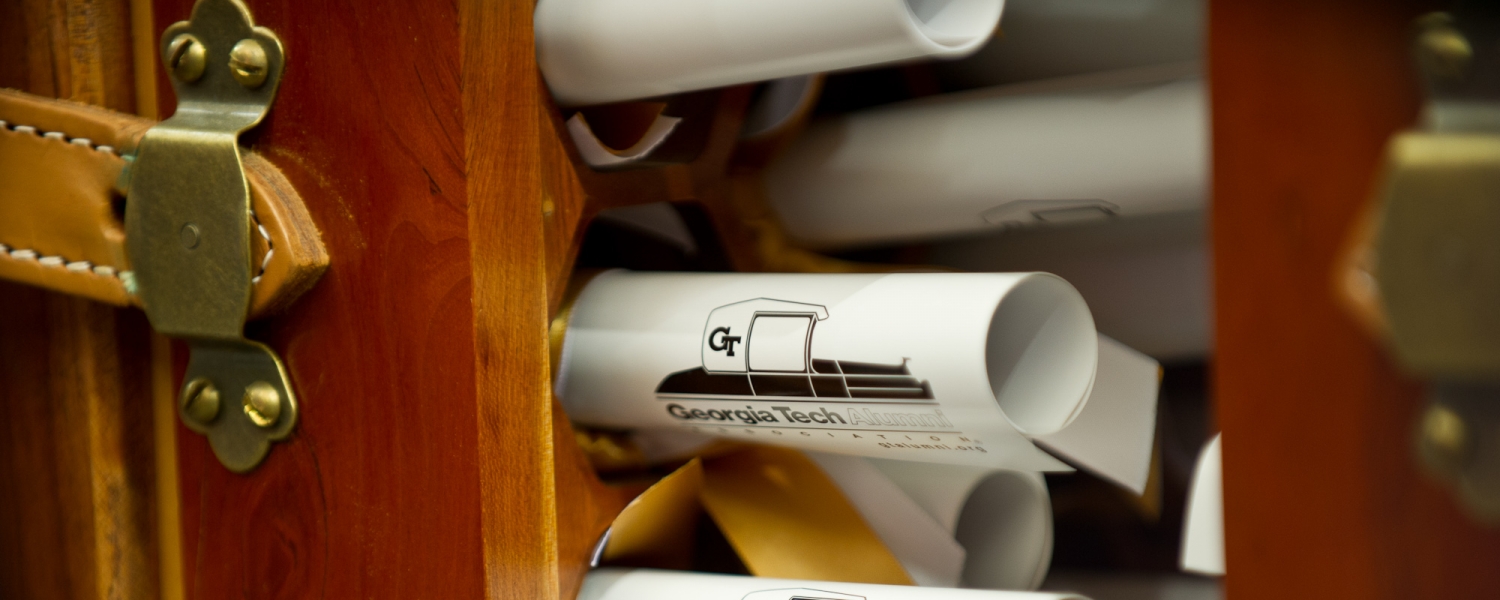
Destin Sandlin uses examples from his YouTube channel "Smarter Every Day" to challenge you to ask questions, consider the science, and explore deeper to see the world differently.
Abstract:
Destin Sandlin uses examples from his YouTube channel "Smarter Every Day" to challenge you to ask questions, consider the science, and explore deeper to see the world differently.
Biography:
Destin Sandlin is an American engineer best known for his educational video series, Smarter Every Day (SED), which is hosted on a YouTube channel of the same name launched in 2007.
Sandlin received his Bachelors of Science from the University of Alabama, where he studied mechanical engineering. Later he obtained a graduate degree in Aerospace Engineering from the University of Alabama in Huntsville.
Sandlin began posting educational videos in 2007, and his first video to reach one million views cleared that milestone on July 10, 2009. The video was about chicken head tracking using chickens that Destin bought for his father as a demonstration. Because of its popularity that video retroactively had the Smarter Every Day label added to it.
Sandlin formally launched Smarter Every Day (SED) On Apr 24, 2011 with a video titled "Detonation vs Deflagration - Smarter Every Day 1, which became the title for subsequent videos and the sole focus of his YouTube channel.
Episodes of Smarter Every Day revolve around scientific exploration and discovery and feature Sandlin as host and narrator. Sandlin is fascinated by flight and space, and his Smarter Every Day video library reflects that.
Event Details
Date/Time:
Abstract:
The Ig Nobel Prizes honor achievements that make people LAUGH, then THINK. Ten new prizes have been awarded every year since 1991, in gala ceremonies at Harvard and MIT, with winners traveling from around the world, and Nobel laureates physically handing out the Ig Nobel Prizes. The whole history of science, technology and medicine is a parade of things that at first made people LAUGH, then THINK. The Igs focuses public curiosity on the early, thought-provoking, "Is it good, bad, or too-early-to-tell?" stage of things.
About Frontiers in Science Lectures
Lectures in this series are intended to inform, engage, and inspire students, faculty, staff, and the public on developments, breakthroughs, and topics of general interest in the sciences and mathematics. Lecturers tailor their talks for nonexpert audiences.
This lecture is co-sponsored with the Georgia Tech School of Public Policy.
**Note Marc Abrahams will have a seminar at 10am in Klaus, Room 1116 East & West.
Event Details
Date/Time:
The title of this talk remains one of the most important and challenging questions in theoretical astrophysics. The explosive deaths of massive stars, core-collapse supernovae, are some of the most energetic events in the Universe; they herald the birth of neutron stars and black holes, are a major site for nucleosynthesis, influence galactic hydrodynamics, trigger further star formation, and are prodigious emitters of neutrinos and gravitational waves.
Though these explosions play an important and multifaceted role in many cosmic phenomena, the details of the explosion mechanism have remained elusive for many decades. The fundamental challenge of core-collapse theory is to understand what makes the difference between a fizzled result and successful explosions. Ultimately, answering this question will require both theory and observational constraints. In this talk, I will present a unifying theory for core-collapse supernova explosions, and I will present our observational constraints on which stars actually explode.
Event Details
Date/Time:
Proteins can adopt a variety of intricate conformations, including native, unfolded, misfolded and aggregated forms. In the latter case, generally 10’s-1000’s of protein molecules bind together to form an aggregate structure that could be random and amorphous or highly specific and well-ordered, such as an amyloid fibril. Amyloid fibril formation of certain proteins is associated with disease, including Alzheimer’s, Parkinson’s, and ALS, wherein the aberrant misfolding and aggregation of a particular protein is central to pathogenesis. Prion protein is notorious for forming infectious amyloid-like species that cause transmissible spongiform encephalopathies, including BSE, CJD and Kuru. In other cases, proteins form amyloid fibrils as part of their natural functional role, forming so-called functional amyloid.
Given the correct conditions, potentially any protein can be induced to form amyloid-like fibrils, also called nanofibrils. Nanofibrils hold promise for applications ranging from biosensors, nanowires, cell scaffolding, and perhaps as food ingredients. Protein nanofibrils have a high aspect ratio (1000’s nm in length, 7-12 nm wide), strength, and surface charge profile that make them attractive for such applications. The mechanisms of protein aggregation must be better understood in order to prevent amyloid formation, in the case of disease-associated amyloid, or such that it can be controlled in the case of engineering functional nanofibrils.
Event Details
Date/Time:
Abstract
There has been tremendous growth in studying nonequilibrium systems of particle assemblies which can exhibit jamming effects in the absence of quenched disorder. Here we examine the dynamics of active and passive particles interacting with random or periodic substrates and obstacle arrays, and show that it is possible to make a clear distinction between jammed systems and clogged systems. Non-active particles flowing through random obstacle arrays reach a clogged state when the particle density is still well below that at which an obstacle free system would jam. The clogged states are spatially heterogeneous, fragile, and have a pronounced memory effect, whereas jammed states are homogeneous, robust, and have much weaker memory effects.
We outline a possible scenario in which jamming is dominated by a diverging length scale associated with a critical density at point J, while clogging is associated with the coarsening of a dense area across the sample. We have also investigated clogging and jamming in active matter or self-motile particle systems, which include biological systems such as run-and-tumble bacteria or crawling cells as well as non-biological systems such as self-driven colloids or artificial swimmers. For active particles driven over random disorder we find that for intermediate amounts of self-motility the system does not clog; however, as the self-propulsion of the particles increases, there is a strong reduction of the mobility due to a self-clogging or self-clustering in the system that resembles the "faster is slower" effect found in certain pedestrian panic models.
Event Details
Date/Time:
Figure 1: Athermal phase separation of active matter. (Adapted from Takatori & Brady PRE (2015).)
A distinguishing feature of many living systems is their ability to move, to self-propel—to be active. Through their motion living systems are able self-assemble: birds flock, fish school, bacteria swarm, etc. But such behavior is not limited to living systems. Recent advances in colloid chemistry have led to the development of synthetic, nonliving particles that are able to undergo autonomous motion by converting chemical energy into mechanical motion and work—chemical swimming.
This swimming or intrinsic activity imparts new behaviors to active matter that distinguish it from equilibrium systems. Active matter generates its own internal pressure (or stress), which can drive it far from equilibrium, and by so doing active matter can control and direct its own behavior and that of its surroundings. In this talk I will discuss our recent work on active matter and on the origin of a new source of stress that is responsible for self-assembly and pattern formation in active matter systems.
Bio - Dr. Brady’s research interests are in the mechanical and transport properties of two-phase materials, especially complex fluids such as biological liquids, colloid dispersions, suspensions, porous media, active matter, etc. His research combines statistical and continuum mechanics to understand how macroscopic behavior emerges from microscale physics. He is the co-inventor of the Stokesian Dynamics technique for simulating the behavior of particles dispersed in a viscous fluid under a wide range of conditions. Recently, Dr. Brady discovered a new organizing principle for active matter known as the swim pressure.Dr. Brady has been recognized for his work by several awards, including a Presidential Young Investigator Award, the Professional Progress Award of the American Institute of Chemical Engineers, the Bingham Medal of the Society of Rheology and the Fluid Dynamics Prize of the American Physical Society. Dr. Brady served as an associate editor of the Journal of Fluid Mechanics and editor of the Journal of Rheology. He is a fellow of the American Physical Society, the American Academy of Arts & Sciences and a member of the National Academy of Engineering.
Event Details
Date/Time:
Complex systems are those systems that are comprised of a large number of interacting units, such as neurons in a brain, and individual animals in fish schools and ant colonies. Each unit acts on its own, using only local information, and there is no centralised control of the collective. The thousands of tiny interactions between the individuals leads to sophisticated ‘emergent’ behaviour at the group level, such as solving mazes, making efficient trade-offs and building self-assembled, adaptive structures. This is often referred to as ‘swarm intelligence’, and offers an alternative approach to the hierarchical, top-down control used in most human-designed systems.
I will outline some of my past and future work in this field, using a combination of field work, lab studies and computational models to uncover how ant colonies and the slime mould Physarum polycephalum use simple behavioural rules to gain the benefits of swarm intelligence. For more information, check out my website: https://chrisrreid.wordpress.com/
Event Details
Date/Time:
The central narrative of contemporary biology is that DNA encodes all relevant information for an organism’s function and form. While this genotype-to-phenotype framing is appealing for its reductionist simplicity, it has a substantial problem. Between nanometer-scale DNA and organismal-scale phenotype sits a gap of 5 to 9 orders of magnitude in length.
This gap covers everything from active protein diffusion, and macromolecular self-assembly, to biopolymer networks and pattern forming mechanical instabilities. In other words, the story of how organisms get their function and form starts with genes, but rapidly transitions to the language of soft matter as we examine larger and longer length scales. In this talk, I’ll address the technological challenges and solutions for understanding multiscale biophysics in an experimental setting.
Along the way, I will discuss how cm-scale cartilage tissue achieves its remarkable mechanical properties through biopolymer self-organization, and how advances in big data and cloud computing can be leveraged for visualizing this nm-scale structure. I will continue to develop the theme of multiscale biophysics in the context of cell-cell fusion, a remarkably common, yet mysterious processes in which individual cells fuse together to increase their size ~1,000-fold while decreasing metabolic costs by ~75%.
I will also briefly touch on current work studying embryonic morphogenesis where cellular growth gradients drive the geometric nonlinearities underlying emergent patterns. The physics of phase transitions, instabilities, and networks will become reoccurring themes that appear in surprising and unexpected ways. In closing the talk, I will discuss how bridging the genotype-to-phenotype gap is broadly pushing new developments in soft matter physics.
Event Details
Date/Time:
Recently proposed short period metamorphic InSbxAs1-x/InSbyAs1-y superlattices (SLs) [1, 2] manifest a new class of quasi 3D materials with ultra-low bandgap. Application of the virtual substrate approach relieves strong limitations dictated by the substrate lattice constant and makes it possible to grow materials with high crystalline quality in the entire range of the alloy compositions.
We present experimental and theoretical analysis of the carrier energy spectrum and band structure parameters of short period metamorphic InSbxAs1-x/InSbyAs1-y SLs with different periods and layer thicknesses. We demonstrate that the SL bandgap measured with the interband magneto-absorption technique can be varied in the range from 120 to 0meV by changing the SL period. The spectral position of the photoluminescence line correlates with the bandgap energy Eg. Cyclotron resonance peak energy in SLs with the E_g≈0 shows square root dependence on the magnetic field in the range from 0 to 16T. This manifests a linear character of the electron dispersion, so the SLs belong to a new class of Dirac semimetals. The calculated energy spectrum in the SL with a zero bandgap is linear in a wide energy range. The Dirac velocity is determined by the material parameters of SL layers and SL design. We present a picture of the modification of the energy spectrum caused by variation of the layer thicknesses in short period SLs. The impacts of dimensional quantization, tunneling and band interaction on the SL band structure as well as vertical hole transport in metamorphic InSbxAs1-x/InSbyAs1-y superlattices will be discussed.
A number of spectacular physical phenomena predicted in Dirac semimetals stimulated an active search of materials with the linear carrier dispersion. It was shown that Hg1-xCdxTe alloy with x=0.17 behaves as a Dirac semimetal with 3D conical bands [3]. The important advantages of metamorphic InSbxAs1-x/InSbyAs1-y superlattices are high design flexibility and the opportunity to control the carrier spectrum by varying the SL period rather than the material composition. The new materials can be easily integrated into more complex semiconductor structures and devices such as p-n junctions, semiconductor-semimetal heterostructures, etc. This opens a new prospect for both fundamental research and applications in areas including nonlinear optics and quantum computing.
- G.Belenky, Y.Lin, L.Shterengas, D.Donetski, G.Kipshidze, S.Suchalkin, Electronic Letters, v. 51(19), 917 (2015).
- W.Sarney, S.Svensson, Y.Lin, D.Donetsky, L.Shterengas, G.Kipshidze, G. Belenky, Journal of Applied Physics, v. 119, 215704 (2016).
- M.Orlita, D.M.Basko, M.S.Zholudev, F.Teppe, W.Knap, V.I.Gavrilenko, N.N.Mikhailov, S.A.Dvoretskii, P.Neugebauer, C.Faugeras, A-L.Barra, G.Martinez, M.Potemski, Nature Physics, v. 10, 223 (2014).
Event Details
Date/Time:
Airborne wind energy (AWE) systems, which replace conventional towers with tethers and lifting bodies, have the potential to unlock vast amounts of untapped energy at altitudes unreachable by towers. However, the dynamic modeling and control design for these tethered systems is far from optimized, and full-scale experimental prototyping costs act as a bottleneck to AWE systems’ widespread adoption.
In response to these challenges, we have developed a first-in-world 1/100-scale platform for characterizing the flight dynamics and closed-loop control of AWE systems. This seminar will show how this 1/100-scale platform, which utilizes a water channel as its flow medium, replicates the dynamic characteristics of the full-scale system under appropriate model and controller scaling laws.
The presentation will examine how the water channel platform has been used for the rapid characterization of a multitude of stationary AWE system designs, along with control design for AWE systems that augment their power production by flying in periodic crosswind motions.
Bio - Chris Vermillion received his Ph.D. in Electrical Engineering from the University of Michigan in 2009 and received his undergraduate degrees in Aerospace and Mechanical Engineering from the University of Michigan in 2004. Immediately following his Ph.D. work, Dr. Vermillion worked on advanced automotive powertrain control, focusing on constrained optimal control approaches that simultaneously addressed the competing performance interests of fuel economy, emissions, drivability, and torque delivery.Subsequently he served as a Lead Engineer for Altaeros Energies and managed all of the dynamic modeling, control system design, software development, and embedded hardware development for Altaeros’ lighter-than-air wind energy system.
Dr. Vermillion has participated in the full-scale fight testing of two of Altaeros’ designs. Dr. Vermillion is currently an Assistant Professor at UNC-Charlotte, where his research focuses on the dynamic characterization, design optimization, and optimal control of airborne wind energy systems, marine hydrokinetic energy systems, and energy-efficient connected and autonomous vehicles. Dr. Vermillion was the recipient of the National Science Foundation’s CAREER Award in 2015.
Event Details
Date/Time:
Pages
